Image-guided and bronchoscopic localization techniques used to facilitate minimally invasive pulmonary metastasectomies
Introduction
The lung is a common site of metastasis for several cancers including breast, colorectal, renal cell, soft tissue sarcoma, and osteosarcoma (1). Over the last several decades, pulmonary metastasectomy has been consistently associated with improved overall survival in well-selected patients (2-5). As with operations for primary lung cancer there has been a shift toward a minimally invasive approach to metastasectomy (6). This poses a challenge as small metastasis deep in the lung parenchyma can be difficult to appreciate without direct bimanual palpation through a thoracotomy.
Several techniques have been developed to facilitate localization of pulmonary metastases during minimal invasive resection using either a video-assisted thoracoscopic surgery (VATS) or a robotic-assisted thoracoscopic surgery (RATS) approach (7). To date, the ideal technique for localization of pulmonary metastasis remains undefined. Herein, we provide a brief review of the indications for minimally invasive pulmonary metastasectomy and in-depth comparison of the effectiveness and safety of each localization method. Our aim is to provide the reader with a focused clinical review of the available techniques and the limitations of the current literature.
Indication for minimally invasive metastasectomy
Indications for minimally invasive pulmonary metastasectomy are the same as for open metastasectomy. They have been expanded from the original criteria proposed by Alexander and Haight in 1947 to include five components (8,9). (I) Suitable patients must have sufficient pulmonary reserve and fitness to tolerate resection. (II) Primary disease must be controlled or controllable. (III) Extrathoracic metastasis must be resectable or previously resected. (IV) Pulmonary metastasectomy should achieve an R0 resection. (V) Operative treatment should represent the best treatment option among current therapeutic modalities. When these criteria are not met then pulmonary metastasectomy should not be performed and alternative treatments should be considered. More recently, other considerations for metastasectomy have been evaluated to help select patients most likely to benefit from pulmonary resection. Together these factors comprise a proposed tumor, node, metastasis (TNM) classification system for pulmonary metastasectomy and include primary tumor activity, nodal involvement, and number of metastasis (10). Additional risk factors for recurrence after pulmonary metastasectomy include age less than 70 and presence of extra-thoracic metastases. The type of primary tumor is also an important consideration (11). The timing of pulmonary metastasis relative to the original date of diagnosis can be considered as well. Synchronous pulmonary metastases are identified at the time of original primary cancer diagnosis. These are associated with a worse prognosis overall than metachronous pulmonary metastases which develop later as a site of progression or recurrence (12). In general, the number of pulmonary metastases should not be increasing in patients where pulmonary resection for disease control is planned. The ongoing appearance of new sites of disease within the lung suggests more diffuse and systemic disease than what is currently visualized on a chest computed tomography (CT) scan and local control of pulmonary disease in these patients is less likely to improve overall survival. Together, these works speak to the need to personalize treatment based on individual risk factors and the biology of tumor.
When a complete resection cannot be performed through a minimally invasive approach than an open thoracotomy should be considered. Traditional thoracotomy remains an excellent option for metastasectomy and in some cases may be the preferred treatment option (13). As reported by Cerfolio et al., bimanual palpation of the lung through thoracotomy may result in discovery of occult nodules in up to 20% of patients (14). However, there is also ample evidence supporting the use of both a VATS and RATS approach to metastasectomy and a minimally invasive approach is preferred in expert consensus (15). In metanalysis, systematic review, and large registry series, a minimally invasive approach has been associated with equivalent disease-free and overall survival (6,16,17). Metastasectomy can be performed on multiple occasions upon the development of new nodules and an initial minimally invasive approach may facilitate additional ipsilateral procedures through reduction of adhesions (18).
Localization techniques
Several techniques have been developed to aid in the intraoperative identification of metastatic nodules during minimally invasive metastasectomy. There is no formal recommendation for when to use a localization technique. However, lesions less than 1 cm in size and more than 5 mm in depth from the pulmonary surface tend to be the most difficult to appreciate in a minimally invasive approach (19). Localization methods fall into three broad categories: (I) intraoperative imaging; (II) image-guided labeling; (III) and systemic targeting (Figure 1). The merits of each of these techniques are discussed in depth below and outlined in Table 1.
Table 1
Method | Mechanism | Advantage | Disadvantage |
---|---|---|---|
Intraoperative imaging | US probe through thoracoscopic port used to identify pulmonary nodules | Real-time imaging of lung | User dependent |
Potential discovery of occult nodules | Limited in emphysema and incomplete lung collapse | ||
Low complication risk | |||
Single invasive procedure | |||
Image-guided labeling | Preoperative image-guided percutaneous or transbronchial labeling of pulmonary nodules | Established technology with significant body of supporting literature | Requires multiple invasive procedures |
Multiple agents used including wires, fiducials, dyes, radiotracer, and ICG | Various methods may be more suited to RATS or VATS approach | Can be resource demanding | |
Can be performed with or without specialized cameras/fluoroscopy | Potential for complications (hemothorax/pneumothorax) | ||
Only enables resection of pre-operatively identified nodules | |||
Systemic targeting | Peripheral infusion or inhalation of ICG prior to resection localizes to pulmonary nodules | Single invasive procedure | May require hospital visit for infusion 24 hours prior to procedure |
Intraoperative imaging using an NIR thoracoscope provides real-time identification of NIR fluorescence | Potential for discovery of occult metastasis | Requires NIR camera | |
Low complication risk | Emerging technology |
US, ultrasound; ICG, indocyanine green; RATS, robotic-assisted thoracoscopic imaging; VATS, video-assisted thoracoscopic imaging; NIR, near infrared.
Intraoperative imaging
Intraoperative imaging for identification of pulmonary metastasis is primarily accomplished through ultrasonography (US). Real-time intraoperative CT is an option but is extremely cumbersome and limited by associated radiation exposure to the patient and operating room staff. Thoracoscopic US of the collapsed lung was popularized in 1990s and has been refined since that time (20-22). It provides visualization of metastatic as well as primary disease, but is more limited when used for ground glass opacities and subsolid nodules (23). During thoracoscopy a 10 mm ultrasound probe is introduced through a VATS port or the assistant port in an RATS approach. The probe is run over the surface of the lung to identify nodules that lie deep within the parenchyma. In comparison to palpation, Matsumoto et al. reported a 12% increase in the number of lesions found using US (24). In a similar comparison, Taurchini reported a 6% increase in occult nodules found with intraoperative US (25). Compared to radio-guided techniques, intraoperative US resulted in a similar number of discovered nodules but with an associated decrease in complications (26).
An additional benefit of intraoperative US is characterization of the visualized nodule which can help differentiate malignancy from benign disease (27). Unlike preoperative localization techniques, intraoperative US provides a survey of the lung parenchyma and can facilitate the discovery of occult nodules not identified on pre-operative imaging. This technique is particularly useful in the pediatric population as it does not expose the patient to an additional radiation dose (28). In adults with extensive emphysema, failure of the lung to fully collapse can limit the utility of this technique as the residual air obscures the ultrasonographic view. Furthermore, thoracoscopic US is user dependent and success depends on the skills and fastidiousness of the surgeon or bedside assistant.
Image-guided labeling
Labeling of pre-operatively imaged nodules is the most common method of localizing for metastasectomy. Fiducials or injectable solution can be placed or instilled in the target nodule percutaneously with CT-guidance or with navigational bronchoscopy (29). Methods compatible with percutaneous CT-guidance include hook wires (30-34), microcoils (34-39), fiducials (40,41), and liquid injectables including dyes [methylene blue, indigo carmine, indocyanine green (ICG)] (42-57), contrast agents (lipiodol) (58-60), and radiotracers [technetium-99m (99mTc)] (61-70) (Figure 2). All these techniques can be performed with navigational bronchoscopy with the exception of hook wires. A transbronchial approach may avoid complications such as pneumothorax, hemothorax, dislodgement/migration, and chest pain. Nevertheless, iatrogenic bronchial injury is still possible. The choice of which modality to use depends on the location of the nodule, preference of the surgeon, and the institutional experience. The various methods of image-guided labeling are compared below and outlined in Table 2.
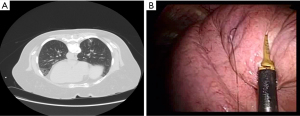
Table 2
Methods | Mechanism | Delivery | Compatibility | Durability | Advantages | Disadvantages | Level of evidence |
---|---|---|---|---|---|---|---|
Hook wire | Visualization of wire with camera | Percutaneous | VATS or RATS | Hours | No specialized equipment | Dislodgement | Case series, prospective trials, and randomized controlled trials |
Higher complication rate | |||||||
Requires same day procedure | |||||||
Microcoil | Fluoroscopy | Percutaneous or navigational bronchoscopy | VATS or RATS but fluoroscopy limited with docked robot | Days | Low complication | Requires fluoroscopy if not combined with ICG | Case series, prospective trials, and randomized controlled trials |
Reduced parenchymal resection | |||||||
Can be combined with ICG | |||||||
Can be staged | |||||||
Gold fiducial | Fluoroscopy | Percutaneous or navigational bronchoscopy | VATS or RATS but fluoroscopy limited with docked robot | Days | Low complication rate | Requires fluoroscopy | Case series |
Can be staged | |||||||
Methylene blue | Visualization of dye with camera | Percutaneous or navigational bronchoscopy | VATS or RATS | Minutes to hours | No specialized equipment | Rapid diffusion | Case series, retrospective cohort studies |
Challenging to localize deeper nodules | |||||||
Inexpensive | |||||||
Indigo carmine/lipiodol | Fluoroscopy and visualization of dye with camera | Percutaneous or navigational bronchoscopy | VATS or RATS but fluoroscopy limited with docked robot | Hours | Visual and fluoroscopic confirmation | Requires fluoroscopy | Case series |
Reduced dispersion | |||||||
Radiotracer (99mTc, 99mTc-MAA) | Detection of radioactivity with gamma probe | Percutaneous or navigational bronchoscopy | VATS or RATS | 36 hours | Hand-held gamma probe can be used with docked robot | User dependence | Case series, prospective trials, retrospective cohort studies |
Can be combined with dyes and other visualization techniques | Special precautions with use of radiotracer | ||||||
ICG | Visualization using near infra-red camera | Percutaneous or navigational bronchoscopy | VATS or RATS | 24 hours alone, several days in combination with microcoils | Can be combined with microcoils | Specialized camera | Case series, prospective studies |
Can disperse and spread | |||||||
Robotic camera with built in near-infrared camera |
VATS, video-assisted thoracoscopic surgery; RATS, robotic-assisted thoracoscopic surgery; ICG, indocyanine green; 99mTc, technetium-99m; MAA, macroaggregated albumin.
Hook wires
Metallic wires can be used for direct site visualization. Under CT guidance, a needle is introduced into or near the pulmonary nodule of interest. A hook wire is then advanced and anchored (Figure 2). Hook wire localization is relatively straightforward and does not require specialized equipment such as infrared camera or fluoroscopy. Success rates range from 91–100%; however, the risk of complications is higher compared to other methods. Pneumothorax is reported in 18–68% of patients (30-34). Most patients are asymptomatic but will occasionally require aspiration or chest tube placement (32,33). Other complications include hematoma, parenchymal hemorrhage, and hemothorax. Hook wire dislodgement or migration can impact wedge resection, but success can still be achieved using the puncture site as guidance (33). Ichinose et al. reports a case of systemic air embolism, but without sequelae and with spontaneous resolution (32).
Microcoils and fiducial markers
The use of microcoils to guide VATS excision of small nodules was piloted by Mayo et al. in 2009 (35). Like hook wires, the fiber-coated microcoils are deployed into the lung parenchyma in or near the nodule through a Chiba needle. When deployed it assumes a tightly coiled ball configuration to anchor it in place. VATS success rates range from 93–100% (34-39). Complications are similar to those of the hook wire technique but occur with lower frequency (34,36). Additionally, resection procedure time was found to be shorter and excised lung volume smaller for microcoils compared to hook wire (34).
Like hook wires and microcoils, percutaneous placement of gold fiducial markers can achieve successful localization for pulmonary metastasectomies. Typically, a 3-mm gold fiducial marker is delivered preoperatively under CT guidance, then identified intraoperatively using fluoroscopy. Reported success rates for this technique are at 98% (40,41). Due to the inert properties of gold and small size of the fiducial, advantages over other metallic markers include patient comfort and flexibility of time between placement and resection. Complications, however, were still reported including pneumothorax, hemoptysis, hematoma, and fiducial migration and embolization (40,41). Finally, all fiducials require use of intraoperative fluoroscopy which can be cumbersome with a docked robotic platform and increase radiation exposure.
Injectable dyes
CT-guided methylene blue dye localization for pulmonary nodules dates back several decades (42-44). The patient is positioned, then 0.3–0.5 mL methylene blue dye is delivered through a Chiba needle into the nodule under local anesthesia with CT guidance. Its distinctive blue color facilitates direct visualization by thoracoscopic vision during VATS.
Methylene blue is advantageous in its simplicity, requiring no special equipment during resection. The localization procedure is short and accurate with success rates as high as 98% (45-47). Kleedehn et al. reported that methylene blue is more efficacious and with less complications when compared to hook wire localization (45). The drawbacks of methylene blue are its rapid diffusion characteristics, risk for spillage, and risk for pneumothorax or hemothorax as with other percutaneous methods. Rapid dye diffusion restricts flexibility and requires same-day operation following dye localization.
Indigo carmine is another blue synthetic dye used for localization, and when coupled with lipiodol, an iodinated oil-based contrast agent, it provides both visual and imaging-based detection. Lipiodol’s radiopacity allows identification by fluoroscopy. The indigo carmine-lipiodol mixture is reported to have a localization success rate of 96–100% (48,49). Complications are related to the risks associated with percutaneous injection.
Lipiodol
Although used in combination with many other agents, lipiodol by itself can be a reliable marker for pulmonary metastatic lesions. Lipiodol is a lipid-soluble contrast medium used for several contrast imaging modalities. Its use has extended to marking pulmonary nodules through fluoroscopy. Localization success rates of 97–99.9% have been reported (58-60). Its advantages are that it does not induce an inflammatory response, identifies nodule depth, and exhibits minimal diffusion. Complications are limited to those attributed to needle insertion as outlined previously, except for extremely rare cases of lipiodol embolization (58).
Radiotracer
The use of 99mTc radiotracer injection was reported by Chella et al. for thoracoscopic resection, with Daniel et al. developing a macroaggregated albumin (MAA) coupled solution that has become the standard (66,71). Preoperatively, 99mTc-labeled radiotracers are injected under CT guidance within the lesion under local anesthesia, typically MAA or colloid (62), same day or the day before. Some centers report injecting 0.1–0.3 mL of nonionic contrast medium (61-64). Intraoperatively, a handheld gamma probe is used to identify areas of increased radioactivity. The maximal radioactivity of the resected nodule can then be compared to residual radioactivity within the parenchyma to ensure compete resection of the injected region.
99mTc radiotracer has a success rate of 95–100% in localizing and resecting lesions (61-72). The marker remains localized at the site of injection up to 24–36 h after injection (53), allowing for flexibility in scheduling operations. Intraoperatively, the handheld gamma probe provides continuous reassessment of location to confirm accurate resection. This technique can be combined with injection of methylene blue to provide an additional visual guidance (72). The procedure is well-tolerated and has minimal risk of complications and morbidity; however, pneumothorax has been reported during radiolabeling (64). Spillage of radiotracer into pleural space is possible, especially if the injection site is near the pleural surface of a major fissure.
Percutaneous ICG
ICG has emerged as a fluorophore for the localization of pulmonary nodules. This near-infrared (NIR) contrast agent is characterized by its ability to emit fluorescence when exposed to specific wavelengths of light, allowing for dynamic imaging of the pulmonary vasculature and nodule localization. A thoracoscopic fluorescence camera allows for immediate detection of ICG during VATS or RATS metastasectomy. The advantages of NIR imaging are its high tissue penetration and low autofluorescence (50).
Reported localization success rates range from 88.6–100% (51-56); a systematic review and meta-analysis by Gkikas et al. [2022] pinned the median reported success rate of CT-guided localization at 94.3% (57). One study used percutaneous ICG as a complementary technique to CT-guided microcoil localization (51). Overall, procedural complications were minimal, with a pneumothorax incidence of 3.4% (52). Minimal diffusion of dye was observed across studies and coupling ICG with albumin further prevented dispersion from the site of injection (53).
Bronchoscopic localization
Endobronchial localization of pulmonary metastases can be achieved using conventional flexible bronchoscopy. However, it is limited by poor access to more peripheral nodules, favoring the advent of newer techniques such as electromagnetic navigation bronchoscopy (ENB), virtual-assisted lung mapping (VAL-MAP) (73), and robotic-assisted bronchoscopy (RAB). After guiding the probe to the target lesion, a number of previously described localization methods can be used, including dyes, lipiodol, and microcoils.
ENB uses the combination of three components: a planning software that converts preoperative CT scans into a virtual three-dimensional (3D) model of the airways, a steerable probe with a position sensor, and an electromagnetic board connected to a computer. This system allows visualization of probe position within the electromagnetic field, which can be used to guide bronchoscopic localization (74). ENB has been used with several marker solutions. Indigo carmine has been studied with a localization success rate of 95.8% (75). Abbas et al. reported a localization success rate of 98.1% with a mixture of iopamidol (a fluorophore), methylene blue, and ICG (76). ICG/iopamidol has also been used comparing ENB with CT-guided percutaneous localization with success rates of 100% and 94.3%, respectively. Its advantages over percutaneous techniques are the lower rates of complications such as pneumothorax and parenchymal hemorrhage, ability to reach lesions not easily accessible percutaneously, and improved patient comfort (77).
Sato et al. expanded on virtual bronchoscopic localization, wherein a 3D virtual reconstruction from thin-slice CT images is used with fluoroscopic dye injection to achieve multiple simultaneous lung markings, the basis of VAL-MAP (73). A prospective, multicenter study with 500 patients who underwent VAL-MAP with indigo carmine marking reported a localization success rate of 91% (78). Detectable marking rates was found to be higher (95.7%) in a study using ICG/indigo carmine dual staining compared to either alone (79).
RAB has emerged as a novel tool for localization of difficult-to-access nodules. Two FDA-approved commercially available systems are currently in use: the electromagnetic Monarch system and shape-sensing Ion system (80). Their use has mainly been piloted for biopsy of pulmonary nodules but has recently expanded to preoperative localization for thoracoscopic surgery. Chan et al. reported a localization success rate of 80% using robotic-RAB for ICG dye-marking in a cone-beam CT hybrid operating room (81), albeit with a small study size of five cases. Bawaadam et al. reported the use of RAB with an ICG-soaked fiducial coil, which allowed fluoroscopic visualization multiple days later, to achieve 100% localization in a series of four patients (Figure 3) (82). Some groups have also described the completion of RAB and RATS under a single anesthetic setting (83). As the technology evolves, patients with metastatic lung lesions can potentially benefit from robotic approaches for metastasectomies.
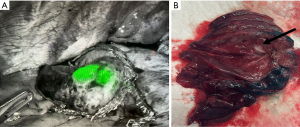
Systemic targeting strategies
Advancements in NIR imaging have led to the development of methods that can localize a nodule by systemically infusing molecular substances intravenously prior to surgery. The molecules accumulate in tumoral regions and have fluorescent tags or innate fluorescent properties that facilitate visual detection with greater ease and accuracy than white light. Inhalation of targeting agents have also been reported. Molecular marking via systemic targeting allows for intraoperative detection of malignant lesions that have been previously identified radiologically as well as radiologically occult disease.
Systemic-ICG targeting
High-dose ICG systemic infusion has been reproducibly shown to preferentially accumulate in multiple types of malignant lesions (84). A dose of 5 mg/kg with NIR imaging 24 h later has been shown to be optimal (85). The mechanism that allows ICG localization to cancer deposits is known as the enhanced permeability and retention effect (86). Increased pressure gradients and abnormally leaky capillaries in the peri-tumoral region facilitates ICG dye accumulation. NIR optical imaging built into thoracoscopes and robotic camera systems detect the fluorescence and allow for identification of known lesions as well as occult tumor deposits. There are minimal side effects and a low toxicity profile to high dose ICG infusion (84,85,87,88). ICG-infusion has been shown to be safe and effective in a pediatric population (89). The main limitation is not size of the lesion but depth from the pleural surface; a depth less than 2 cm is favorable (85,87). In addition to systemic infusion, Wang et al. reports ICG inhalation as a feasible and safe method for detection, successfully localizing 88% of studied pulmonary nodules (90). More cohort studies are needed to evaluate the efficacy of inhaled agents in comparison with other localization techniques.
Specific targeting molecules
Investigators have developed more specific molecules linked with fluorescent tracers that can be delivered systemically to aid in nodule detection using NIR optical cameras. Pafolacianine is a folate analogue with an ICG-like conjugate that binds to folate receptors that are present on approximately 85% of pulmonary malignancies. This molecule is infused between 1–24 h prior to surgery and exhibits mild infusion reactions and no serious adverse events (91). The advantage of this molecule is that it has less autofluorescence than ICG and a better depth of penetration for detection, although a false positive rate of 15–25% was reported in the ELUCIDATE trial (91). The molecule was shown to identify numerous tumor types including colorectal, breast, renal, melanoma, spine, ovarian, prostate, sarcomatous, and neuroendocrine histologies. Another folate receptor targeting molecule known as OTL38 demonstrated ability to optically fluoresce osteosarcoma deposits (86).
Monoclonal antibodies have been combined with NIR probes to facilitate the intraoperative identification of pulmonary nodules. Colorectal pulmonary metastatic lesions were enhanced with SMG-101, a tracer that combines a monoclonal antibody to carcinoembryonic antigen (CEA) with a NIR fluorescent tracer (92). This targeting molecule was successful at fluorescing colorectal pulmonary metastatic deposits but was similarly limited by depth of penetration. In a murine model, a HER2/NEU antibody conjugated with a fluorescing tracer was shown to accurately identify pulmonary nodules expressing HER2/NEU (93).
In an effort to increase the accuracy of identifying malignant targets, a pH-activated NIR fluorescent nanoprobe consisting of a dye IR780 conjugated with calcium phosphate has been studied (89). This compound takes advantage of the extracellular acidity common to solid tumors where the conjugate breaks down in the acidic environment enhancing differentiation between normal tissue and tumor (94).
The advancements in NIR optical technology and synthesis of fluorescing agents will likely continue to increase a surgeon’s ability to visually identify metastatic and primary lung lesions without requiring an initial invasive procedure such as percutaneous or bronchoscopic labelling.
Emerging technologies
In addition to the more established techniques described above, there are emerging technologies being evaluated in animal models and early pilot studies. This includes augmented reality and navigational systems, in which pre-operative CT-scans and localization markers are used to generate a 3D projection of the lung and an associated nodule, which can be viewed on a surgeon worn headset during the resection (95). This technology has shown promise in animal models and human trial with a 70% success rate (96). It has the advantage of streamlining the localization process but its cost and benefit over existing technologies has not yet been evaluated.
The use of artificial intelligence is also being evaluated. This technology is already used in the radiologic identification of pulmonary nodules, but may soon be available for intraoperative assessment (97,98). Broadly, this technology can aid surgeons by predicting intraoperative pathology and helping ensure adequate margins (98). It has been used in neurosurgery and endocrine surgery to help identify residual malignancy and the likelihood for nodal metastasis (99-101). The introduction of artificial intelligence to thoracic surgery is likely eminent, although the exact manner in which it will be employed is uncertain.
Critical assessment of the literature
To date, there are more than a dozen described techniques for localizing pleural metastasis that have been used in conjunction with minimally invasive metastasectomy. The reported effectiveness of these techniques is generally >95%. This leads to the dilemma: what is the optimal localization technique? To answer this question, it is important to note the context of these studies. While thorough and well performed, the vast majority of studies represent case reports, small case series, and retrospective cohort studies. They are often heterogenous in nature and include primary lung cancer, metastatic disease, and indeterminate ground glass opacities. Despite the best intentions of the authors there is undoubtedly an element of selection bias. Metanalysis has been performed for more established techniques like hook-wire, microcoil, and lipiodol injection as well as ICG localization, but it remains difficult to compare between these varied approaches (7,57). There are randomized trials evaluating individual techniques against standard light detection, but robust studies comparing disparate methods are lacking. A further concern is cost with little data to help guide the surgeon or hospital administration.
Given these limitations, the choice of localization remains at the discretion of the surgeon and their institutional practice. As with many surgical techniques, surgeon comfort/familiarity with a particular procedure may be the greatest driver of success. The authors favor use of radiotracer as it is easy to adapt to both a RATS or VATS approach. Alternatively, if the approach is solely RATS then ICG soaked microcoils can be extremely effective and may be performed as a single or staged procedure (82). Given the growing acceptance of systemic techniques, head-to-head trials including cost analysis will be of particular value.
Conclusions
The decision to proceed with minimally invasive pulmonary metastasectomy should be based on a patient’s individual risk factors, likelihood of successful local control, absence of other sites of systemic disease, and tumor biology. Minimally invasive pulmonary metastasectomy in conjunction with localization procedures is associated with a >95% success rate. The choice of localization technique is at the discretion of the surgeon and their institutional practice. Understanding the advantages and disadvantages of different methods can help guide the thoracic surgeon towards better patient outcomes based on the available resources. Direct comparison of systemic targeting and traditional image-guided labeling through randomized trials is needed to determine the optimal modality.
Acknowledgments
Funding: None.
Footnote
Provenance and Peer Review: This article was commissioned by the Guest Editors (Anthony W. Kim and Takashi Harano) for the series “The Role of Minimally Invasive Approaches in the Pulmonary Oligometastases” published in Video-Assisted Thoracic Surgery. The article has undergone external peer review.
Peer Review File: Available at https://vats.amegroups.com/article/view/10.21037/vats-23-80/prf
Conflicts of Interest: All authors have completed the ICMJE uniform disclosure form (available at https://vats.amegroups.com/article/view/10.21037/vats-23-80/coif). The series “The Role of Minimally Invasive Approaches in the Pulmonary Oligometastases” was commissioned by the editorial office without any funding or sponsorship. G.A.W.’s research is funded in part by the Yale Cancer Center, #IRG 17-172-57 from the American Cancer Society, a Career Enhancement Program Grant from the Yale SPORE in Lung Cancer (P50 CA196530) and by the International Lung Cancer Foundation. G.A.W. also received consulting fees from Advisory board for AstraZeneca. The authors have no other conflicts of interest to declare.
Ethical Statement: The authors are accountable for all aspects of the work in ensuring that questions related to the accuracy or integrity of any part of the work are appropriately investigated and resolved.
Open Access Statement: This is an Open Access article distributed in accordance with the Creative Commons Attribution-NonCommercial-NoDerivs 4.0 International License (CC BY-NC-ND 4.0), which permits the non-commercial replication and distribution of the article with the strict proviso that no changes or edits are made and the original work is properly cited (including links to both the formal publication through the relevant DOI and the license). See: https://creativecommons.org/licenses/by-nc-nd/4.0/.
References
- Davidson RS, Nwogu CE, Brentjens MJ, et al. The surgical management of pulmonary metastasis: current concepts. Surg Oncol 2001;10:35-42. [Crossref] [PubMed]
- Pastorino U, Buyse M, Friedel G, et al. Long-term results of lung metastasectomy: prognostic analyses based on 5206 cases. J Thorac Cardiovasc Surg 1997;113:37-49. [Crossref] [PubMed]
- Van Raemdonck D, Friedel G. The European Society of Thoracic Surgeons lung metastasectomy project. J Thorac Oncol 2010;5:S127-9. [Crossref] [PubMed]
- Milosevic M, Edwards J, Tsang D, et al. Pulmonary Metastasectomy in Colorectal Cancer: updated analysis of 93 randomized patients - control survival is much better than previously assumed. Colorectal Dis 2020;22:1314-24. [Crossref] [PubMed]
- Stamenovic D, Hohenberger P, Roessner E. Pulmonary metastasectomy in soft tissue sarcomas: a systematic review. J Thorac Dis 2021;13:2649-60. [Crossref] [PubMed]
- Gonzalez M, Brunelli A, Szanto Z, et al. Report from the European Society of Thoracic Surgeons database 2019: current surgical practice and perioperative outcomes of pulmonary metastasectomy. Eur J Cardiothorac Surg 2021;59:996-1003. [Crossref] [PubMed]
- Park CH, Han K, Hur J, et al. Comparative Effectiveness and Safety of Preoperative Lung Localization for Pulmonary Nodules: A Systematic Review and Meta-analysis. Chest 2017;151:316-28. [Crossref] [PubMed]
- Alexander J, Haight C. Pulmonary resection for solitary metastatic sarcomas and carcinomas. Surg Gynecol Obstet 1947;85:129-46. [PubMed]
- Sudarshan M, Murthy SC. Current Indications for Pulmonary Metastasectomy. Surg Oncol Clin N Am 2020;29:673-83. [Crossref] [PubMed]
- Migliore M, Gonzalez M. TNM classification for lung metastases. Video-assist Thorac Surg 2021;6:36. [Crossref]
- Forster C, Ojanguren A, Perentes JY, et al. Personalized approach for video-assisted thoracic surgery lung metastasectomy. Video-assist Thorac Surg 2020;5:22. [Crossref]
- Loukeri AA, Kampolis CF, Ntokou A, et al. Metachronous and synchronous primary lung cancers: diagnostic aspects, surgical treatment, and prognosis. Clin Lung Cancer 2015;16:15-23. [Crossref] [PubMed]
- Gao E, Li Y, Zhao W, et al. Necessity of thoracotomy in pulmonary metastasis of osteosarcoma. J Thorac Dis 2019;11:3578-83. [Crossref] [PubMed]
- Cerfolio RJ, Bryant AS, McCarty TP, et al. A prospective study to determine the incidence of non-imaged malignant pulmonary nodules in patients who undergo metastasectomy by thoracotomy with lung palpation. Ann Thorac Surg 2011;91:1696-700; discussion 1700-1. [Crossref] [PubMed]
- Handy JR, Bremner RM, Crocenzi TS, et al. Expert Consensus Document on Pulmonary Metastasectomy. Ann Thorac Surg 2019;107:631-49. [Crossref] [PubMed]
- Meng D, Fu L, Wang L, et al. Video-assisted thoracoscopic surgery versus open thoracotomy in pulmonary metastasectomy: a meta-analysis of observational studies. Interact Cardiovasc Thorac Surg 2016;22:200-6. [Crossref] [PubMed]
- Numan RC, Baas P, Klomp HM, et al. Optimal surgical management of pulmonary metastases: VATS versus thoracotomy. Respirology 2016;21:188-90. [Crossref] [PubMed]
- Mills AC, Hofstetter WL, Mehran RJ, et al. Repeated Pulmonary Metastasectomy: Third Operations and Beyond. Ann Thorac Surg 2023;115:679-85. [Crossref] [PubMed]
- Suzuki K, Nagai K, Yoshida J, et al. Video-assisted thoracoscopic surgery for small indeterminate pulmonary nodules: indications for preoperative marking. Chest 1999;115:563-8. [Crossref] [PubMed]
- Gossot D, Colomer S, Fourquier P, et al. Thoracoscopic ultrasonic localisation of lung nodules: initial results of an in-vitro and in-vivo study. Endosc Surg Allied Technol 1994;2:153-5. [PubMed]
- Greenfield AL, Steiner RM, Liu JB, et al. Sonographic guidance for the localization of peripheral pulmonary nodules during thoracoscopy. AJR Am J Roentgenol 1997;168:1057-60. [Crossref] [PubMed]
- Santambrogio R, Montorsi M, Bianchi P, et al. Intraoperative ultrasound during thoracoscopic procedures for solitary pulmonary nodules. Ann Thorac Surg 1999;68:218-22. [Crossref] [PubMed]
- Huang YH, Chen KC, Chen JS. Ultrasound for intraoperative localization of lung nodules during thoracoscopic surgery. Ann Transl Med 2019;7:37. [Crossref] [PubMed]
- Matsumoto S, Hirata T, Ogawa E, et al. Ultrasonographic evaluation of small nodules in the peripheral lung during video-assisted thoracic surgery (VATS). Eur J Cardiothorac Surg 2004;26:469-73. [Crossref] [PubMed]
- Taurchini M, Quarato CMI, Frongillo EM, et al. Intraoperative Lung Ultrasound (ILU) for the Assessment of Pulmonary Nodules. Diagnostics (Basel) 2021;11:1691. [Crossref] [PubMed]
- Sortini D, Feo CV, Carcoforo P, et al. Thoracoscopic localization techniques for patients with solitary pulmonary nodule and history of malignancy. Ann Thorac Surg 2005;79:258-62; discussion 262. [Crossref] [PubMed]
- Sperandeo M, Frongillo E, Dimitri LMC, et al. Video-assisted thoracic surgery ultrasound (VATS-US) in the evaluation of subpleural disease: preliminary report of a systematic study. J Ultrasound 2020;23:105-12. [Crossref] [PubMed]
- Gow KW, Saad DF, Koontz C, et al. Minimally invasive thoracoscopic ultrasound for localization of pulmonary nodules in children. J Pediatr Surg 2008;43:2315-22. [Crossref] [PubMed]
- Udelsman BV, Blasberg JD. Advances in Surgical Techniques for Lung Cancer. Hematol Oncol Clin North Am 2023;37:489-97. [Crossref] [PubMed]
- Klinkenberg TJ, Dinjens L, Wolf RFE, et al. CT-guided percutaneous hookwire localization increases the efficacy and safety of VATS for pulmonary nodules. J Surg Oncol 2017;115:898-904. [Crossref] [PubMed]
- Chen YR, Yeow KM, Lee JY, et al. CT-guided hook wire localization of subpleural lung lesions for video-assisted thoracoscopic surgery (VATS). J Formos Med Assoc 2007;106:911-8. [Crossref] [PubMed]
- Ichinose J, Kohno T, Fujimori S, et al. Efficacy and complications of computed tomography-guided hook wire localization. Ann Thorac Surg 2013;96:1203-8. [Crossref] [PubMed]
- Hanauer M, Perentes JY, Krueger T, et al. Pre-operative localization of solitary pulmonary nodules with computed tomography-guided hook wire: report of 181 patients. J Cardiothorac Surg 2016;11:5. [Crossref] [PubMed]
- Hwang S, Kim TG, Song YG. Comparison of hook wire versus coil localization for video-assisted thoracoscopic surgery. Thorac Cancer 2018;9:384-9. [Crossref] [PubMed]
- Mayo JR, Clifton JC, Powell TI, et al. Lung nodules: CT-guided placement of microcoils to direct video-assisted thoracoscopic surgical resection. Radiology 2009;250:576-85. [Crossref] [PubMed]
- Rostambeigi N, Scanlon P, Flanagan S, et al. CT Fluoroscopic-Guided Coil Localization of Lung Nodules prior to Video-Assisted Thoracoscopic Surgical Resection Reduces Complications Compared to Hook Wire Localization. J Vasc Interv Radiol 2019;30:453-9. [Crossref] [PubMed]
- Hajjar W, Al-Nassar S, Almousa O, et al. Thoracoscopic resection of suspected metastatic pulmonary nodules after microcoil localization technique: a prospective study. J Cardiovasc Surg (Torino) 2017;58:606-12. [Crossref] [PubMed]
- Lee MO, Jin SY, Lee SK, et al. Video-assisted thoracoscopic surgical wedge resection using multiplanar computed tomography reconstruction-fluoroscopy after CT guided microcoil localization. Thorac Cancer 2021;12:1721-5. [Crossref] [PubMed]
- Donahoe LL, Nguyen ET, Chung TB, et al. CT-guided microcoil VATS resection of lung nodules: a single-centre experience and review of the literature. J Thorac Dis 2016;8:1986-94. [Crossref] [PubMed]
- McDermott S, Frenk NE, Fintelmann FJ, et al. Preoperative CT-guided Fiducial Marker Placement for Surgical Localization of Pulmonary Nodules. Radiol Cardiothorac Imaging 2022;4:e210194. [Crossref] [PubMed]
- Sancheti MS, Lee R, Ahmed SU, et al. Percutaneous fiducial localization for thoracoscopic wedge resection of small pulmonary nodules. Ann Thorac Surg 2014;97:1914-8; discussion 1919. [Crossref] [PubMed]
- Lenglinger FX, Schwarz CD, Artmann W. Localization of pulmonary nodules before thoracoscopic surgery: value of percutaneous staining with methylene blue. AJR Am J Roentgenol 1994;163:297-300. [Crossref] [PubMed]
- Vandoni RE, Cuttat JF, Wicky S, et al. CT-guided methylene-blue labelling before thoracoscopic resection of pulmonary nodules. Eur J Cardiothorac Surg 1998;14:265-70. [Crossref] [PubMed]
- Wicky S, Mayor B, Cuttat JF, et al. CT-guided localizations of pulmonary nodules with methylene blue injections for thoracoscopic resections. Chest 1994;106:1326-8. [Crossref] [PubMed]
- Kleedehn M, Kim DH, Lee FT, et al. Preoperative Pulmonary Nodule Localization: A Comparison of Methylene Blue and Hookwire Techniques. AJR Am J Roentgenol 2016;207:1334-9. [Crossref] [PubMed]
- Lin CY, Chang CC, Huang LT, et al. Computed Tomography-Guided Methylene Blue Localization: Single vs. Multiple Lung Nodules. Front Med (Lausanne) 2021;8:661956. [Crossref] [PubMed]
- Karaçam V, Mutlu F, Kaya İ, et al. Feasibility of uniportal VATS in the histopathological diagnosis of the peripheral pulmonary nodules which are marked by methylene blue before resection. Current Thoracic Surgery 2023. doi:
10.26663/cts.2023.0011 .10.26663/cts.2023.0011 - Hasegawa T, Kuroda H, Sato Y, et al. The Utility of Indigo Carmine and Lipiodol Mixture for Preoperative Pulmonary Nodule Localization before Video-Assisted Thoracic Surgery. J Vasc Interv Radiol 2019;30:446-52. [Crossref] [PubMed]
- Matsui T, Takahashi Y, Nakada T, et al. Preoperative percutaneous needle indigo carmine and lipiodol mixture marking in lung segmentectomy. Eur J Cardiothorac Surg 2022;62:ezac432. [Crossref] [PubMed]
- Schaafsma BE, Mieog JS, Hutteman M, et al. The clinical use of indocyanine green as a near-infrared fluorescent contrast agent for image-guided oncologic surgery. J Surg Oncol 2011;104:323-32. [Crossref] [PubMed]
- Ujiie H, Kato T, Hu HP, et al. A novel minimally invasive near-infrared thoracoscopic localization technique of small pulmonary nodules: A phase I feasibility trial. J Thorac Cardiovasc Surg 2017;154:702-11. [Crossref] [PubMed]
- Chang CJ, Lu CH, Gao X, et al. Safety and Efficacy of Cone-Beam Computed Tomography-Guided Lung Tumor Localization with a Near-Infrared Marker: A Retrospective Study of 175 Patients. Life (Basel) 2022;12:494. [Crossref] [PubMed]
- Voulaz E, Giudici VM, Lanza E, et al. Percutaneous Computed Tomography (CT)-Guided Localization with Indocyanine Green for the Thoracoscopic Resection of Small Pulmonary Nodules. J Clin Med 2023;12:6149. [Crossref] [PubMed]
- Zhang C, Lin H, Fu R, et al. Application of indocyanine green fluorescence for precision sublobar resection. Thorac Cancer 2019;10:624-30. [Crossref] [PubMed]
- Polites SF, Fahy AS, Sunnock WA, et al. Use of radiotracer labeling of pulmonary nodules to facilitate excisional biopsy and metastasectomy in children with solid tumors. J Pediatr Surg 2018;53:1369-73. [Crossref] [PubMed]
- Whitlock RS, Patel KR, Yang T, et al. Pathologic correlation with near infrared-indocyanine green guided surgery for pediatric liver cancer. J Pediatr Surg 2022;57:700-10. [Crossref] [PubMed]
- Gkikas A, Lampridis S, Patrini D, et al. How effective is indocyanine green (ICG) in localization of malignant pulmonary nodules? A systematic review and meta-analysis. Front Surg 2022;9:967897. [Crossref] [PubMed]
- Ito K, Shimada J, Shimomura M, et al. Safety and reliability of computed tomography-guided lipiodol marking for undetectable pulmonary lesions. Interact Cardiovasc Thorac Surg 2020;30:546-51. [Crossref] [PubMed]
- Kawanaka K, Nomori H, Mori T, et al. Marking of small pulmonary nodules before thoracoscopic resection: injection of lipiodol under CT-fluoroscopic guidance. Acad Radiol 2009;16:39-45. [Crossref] [PubMed]
- Mogi A, Yajima T, Tomizawa K, et al. Video-Assisted Thoracoscopic Surgery after Preoperative CT-Guided Lipiodol Marking of Small or Impalpable Pulmonary Nodules. Ann Thorac Cardiovasc Surg 2015;21:435-9. [Crossref] [PubMed]
- Galetta D, Bellomi M, Grana C, et al. Radio-Guided Localization and Resection of Small or Ill-Defined Pulmonary Lesions. Ann Thorac Surg 2015;100:1175-80. [Crossref] [PubMed]
- Galetta D, Rampinelli C, Funicelli L, et al. Computed Tomography-Guided Percutaneous Radiotracer Localization and Resection of Indistinct/Small Pulmonary Lesions. Ann Thorac Surg 2019;108:852-8. [Crossref] [PubMed]
- Starnes SL, Wolujewicz M, Guitron J, et al. Radiotracer localization of nonpalpable pulmonary nodules: A single-center experience. J Thorac Cardiovasc Surg 2018;156:1986-92. [Crossref] [PubMed]
- Manca G, Davini F, Tardelli E, et al. Clinical Impact of Radioguided Localization in the Treatment of Solitary Pulmonary Nodule: A 20-Year Retrospective Analysis. Clin Nucl Med 2018;43:317-22. [Crossref] [PubMed]
- Ambrogi MC, Melfi F, Zirafa C, et al. Radio-guided thoracoscopic surgery (RGTS) of small pulmonary nodules. Surg Endosc 2012;26:914-9. [Crossref] [PubMed]
- Chella A, Lucchi M, Ambrogi MC, et al. A pilot study of the role of TC-99 radionuclide in localization of pulmonary nodular lesions for thoracoscopic resection. Eur J Cardiothorac Surg 2000;18:17-21. [Crossref] [PubMed]
- Doncic N, Zech CJ, Wild D, et al. CT-guided percutaneous marking of small pulmonary nodules with [99mTc]Tc-Macrosalb is very accurate and allows minimally invasive lung-sparing resection: a single-centre quality control. Eur J Nucl Med Mol Imaging 2023; Epub ahead of print. [Crossref] [PubMed]
- Grogan EL, Jones DR, Kozower BD, et al. Identification of small lung nodules: technique of radiotracer-guided thoracoscopic biopsy. Ann Thorac Surg 2008;85:S772-7. [Crossref] [PubMed]
- Stiles BM, Altes TA, Jones DR, et al. Clinical experience with radiotracer-guided thoracoscopic biopsy of small, indeterminate lung nodules. Ann Thorac Surg 2006;82:1191-6; discussion 1196-7. [Crossref] [PubMed]
- Vollmer I, Sánchez-Izquierdo N, Martínez D, et al. Role of a portable gamma-camera with optical view for margins assessment of pulmonary nodules resected by radioguided surgery. Eur J Nucl Med Mol Imaging 2021;49:361-70. [Crossref] [PubMed]
- Daniel TM, Altes TA, Rehm PK, et al. A novel technique for localization and excisional biopsy of small or ill-defined pulmonary lesions. Ann Thorac Surg 2004;77:1756-62; discussion 1762. [Crossref] [PubMed]
- Nardini M, Bilancia R, Paul I, et al. (99m)Technetium and methylene blue guided pulmonary nodules resections: preliminary British experience. J Thorac Dis 2018;10:1015-21. [Crossref] [PubMed]
- Sato M, Omasa M, Chen F, et al. Use of virtual assisted lung mapping (VAL-MAP), a bronchoscopic multispot dye-marking technique using virtual images, for precise navigation of thoracoscopic sublobar lung resection. J Thorac Cardiovasc Surg 2014;147:1813-9. [Crossref] [PubMed]
- Gildea TR, Mazzone PJ, Karnak D, et al. Electromagnetic navigation diagnostic bronchoscopy: a prospective study. Am J Respir Crit Care Med 2006;174:982-9. [Crossref] [PubMed]
- Hyun K, Park IK, Song JW, et al. Electromagnetic navigation bronchoscopic dye marking for localization of small subsolid nodules: Retrospective observational study. Medicine (Baltimore) 2019;98:e14831. [Crossref] [PubMed]
- Abbas A, Kadakia S, Ambur V, et al. Intraoperative electromagnetic navigational bronchoscopic localization of small, deep, or subsolid pulmonary nodules. J Thorac Cardiovasc Surg 2017;153:1581-90. [Crossref] [PubMed]
- Yang YL, Li ZZ, Huang WC, et al. Electromagnetic navigation bronchoscopic localization versus percutaneous CT-guided localization for thoracoscopic resection of small pulmonary nodules. Thorac Cancer 2021;12:468-74. [Crossref] [PubMed]
- Sato M, Kuwata T, Yamanashi K, et al. Safety and reproducibility of virtual-assisted lung mapping: a multicentre study in Japan. Eur J Cardiothorac Surg 2017;51:861-8. [Crossref] [PubMed]
- Yanagiya M, Sato M, Ijiri N, et al. Virtual-assisted lung mapping using dual staining with indocyanine green and indigo carmine enhanced marking detectability. J Thorac Dis 2022;14:1061-9. [Crossref] [PubMed]
- McLoughlin KC, Bott MJ. Robotic Bronchoscopy for the Diagnosis of Pulmonary Lesions. Thorac Surg Clin 2023;33:109-16. [Crossref] [PubMed]
- Chan JWY, Chang ATC, Yu PSY, et al. Robotic Assisted-Bronchoscopy With Cone-Beam CT ICG Dye Marking for Lung Nodule Localization: Experience Beyond USA. Front Surg 2022;9:943531. [Crossref] [PubMed]
- Bawaadam H, Benn BS, Colwell EM, et al. Lung nodule marking with ICG dye-soaked coil facilitates localization and delayed surgical resection. Ann Thorac Surg 2023; Epub ahead of print. [Crossref]
- Patel PP, Duong DK, Mahajan AK, et al. Single Setting Robotic Lung Nodule Diagnosis and Resection. Thorac Surg Clin 2023;33:233-44. [Crossref] [PubMed]
- Keating J, Newton A, Venegas O, et al. Near-Infrared Intraoperative Molecular Imaging Can Locate Metastases to the Lung. Ann Thorac Surg 2017;103:390-8. [Crossref] [PubMed]
- Predina JD, Newton AD, Corbett C, et al. Near-infrared intraoperative imaging for minimally invasive pulmonary metastasectomy for sarcomas. J Thorac Cardiovasc Surg 2019;157:2061-9. [Crossref] [PubMed]
- Predina JD, Newton A, Deshpande C, et al. Utilization of targeted near-infrared molecular imaging to improve pulmonary metastasectomy of osteosarcomas. J Biomed Opt 2018;23:1-4. [Crossref] [PubMed]
- Predina JD, Newton A, Kennedy G, et al. Near-Infrared Intraoperative Imaging Can Successfully Identify Malignant Pleural Mesothelioma After Neoadjuvant Chemotherapy. Mol Imaging 2017;16:1536012117723785. [Crossref] [PubMed]
- Azari F, Kennedy GT, Zhang K, et al. Impact of Intraoperative Molecular Imaging after Fluorescent-Guided Pulmonary Metastasectomy for Sarcoma. J Am Coll Surg 2022;234:748-58. [Crossref] [PubMed]
- Abdelhafeez AH, Mothi SS, Pio L, et al. Feasibility of indocyanine green-guided localization of pulmonary nodules in children with solid tumors. Pediatr Blood Cancer 2023;70:e30437. [Crossref] [PubMed]
- Wang Z, Tian X, Yang F, et al. Indocyanine green inhalation visualizes lung tumour during video-assisted thoracoscopic surgery. Interdiscip Cardiovasc Thorac Surg 2023;36:ivad071. [Crossref] [PubMed]
- Sarkaria IS, Martin LW, Rice DC, et al. Pafolacianine for intraoperative molecular imaging of cancer in the lung: The ELUCIDATE trial. J Thorac Cardiovasc Surg 2023;166:e468-e478. [Crossref] [PubMed]
- Meijer RPJ, Galema HA, Faber RA, et al. Intraoperative molecular imaging of colorectal lung metastases with SGM-101: a feasibility study. Eur J Nucl Med Mol Imaging 2023; Epub ahead of print. [Crossref] [PubMed]
- Koyama Y, Hama Y, Urano Y, et al. Spectral fluorescence molecular imaging of lung metastases targeting HER2/neu. Clin Cancer Res 2007;13:2936-45. [Crossref] [PubMed]
- Jia Q, Zhang R, Yan H, et al. An Activatable Near-Infrared Fluorescent Probe for Precise Detection of the Pulmonary Metastatic Tumors: A Traditional Molecule Having a Stunning Turn. Angew Chem Int Ed Engl 2023;62:e202313420. [Crossref] [PubMed]
- Li C, Ji A, Jian Z, et al. Augmented reality navigation-guided intraoperative pulmonary nodule localization: a pilot study. Transl Lung Cancer Res 2023;12:1728-37. [Crossref] [PubMed]
- Li C, Zheng Y, Yuan Y, et al. Augmented reality navigation-guided pulmonary nodule localization in a canine model. Transl Lung Cancer Res 2021;10:4152-60. [Crossref] [PubMed]
- de Margerie-Mellon C, Chassagnon G. Artificial intelligence: A critical review of applications for lung nodule and lung cancer. Diagn Interv Imaging 2023;104:11-7. [Crossref] [PubMed]
- Navarrete-Welton AJ, Hashimoto DA. Current applications of artificial intelligence for intraoperative decision support in surgery. Front Med 2020;14:369-81. [Crossref] [PubMed]
- Ilunga-Mbuyamba E, Lindner D, Avina-Cervantes JG, et al. Fusion of Intraoperative 3D B-mode and Contrast-Enhanced Ultrasound Data for Automatic Identification of Residual Brain Tumors. Appl Sci 2017;7:415. [Crossref]
- Wan S, Sun S, Bhattacharya S, et al. Towards an Efficient Computational Framework for Guiding Surgical Resection through Intra-operative Endo-microscopic Pathology. In: Navab N, Hornegger J, Wells W, et al. editors. Medical Image Computing and Computer-Assisted Intervention -- MICCAI 2015. Cham: Springer International Publishing; 2015:421-9.
- Hou F, Yang Z, Gu W, et al. Automatic identification of metastatic lymph nodes in OCT images. In: Optical Coherence Tomography and Coherence Domain Optical Methods in Biomedicine XXIII. SPIE; 2019:173-8.
Cite this article as: Wang A, Rosenberg GM, Woodard GA, Dhanasopon A, Udelsman BV. Image-guided and bronchoscopic localization techniques used to facilitate minimally invasive pulmonary metastasectomies. Video-assist Thorac Surg 2024;9:19.