Lung nodule localization techniques in minimally invasive and video-assisted thoracoscopic surgery: a literature review
Introduction
Background
Lung cancer is one of the most common malignancies worldwide (1). With the advancement in imaging technology and increasing awareness of the early detection of lung cancer, low-dose computed tomography (LDCT) screening has become a commonality (2). The new imaging technology offers better spatial resolution and superior sensitivity in detecting pulmonary nodules with smaller size and sub-solid consistency. This allows for the timely detection of subcentimeter ground-glass nodules (GGNs) which may carry up to a 50% risk of malignancy (3). Due to the potential risk of malignancy hidden within these GGN, many of them warrant further histological investigation via percutaneous biopsy or surgical resection. Ever since the success of laparoscopic abdominal surgery in the 1980s, minimally invasive surgery (MIS) and video-assisted thoracoscopic surgery (VATS) were popularized for a broad spectrum of intrathoracic procedures, especially in resection of small lung lesion, due to their benefits of shorter length of hospital stay and shorter duration of postoperative chest drain placement (4); lesser postoperative wound pain and analgesic requirements; and excellent oncological outcomes in terms of survival compared to conventional thoracotomy (5-8).
However, reducing the invasiveness of thoracic surgery results in increased technical difficulty in performing the surgery and localizing the nodules due to due to their size, location, depth, and consistency (9,10). These issues are particularly prominent in major lung resections for potential malignancies, as complete tumor resection with an adequate resection margin is essential for improving survival and oncological clearance. Inaccurate lung nodule localization leads to conversion to open surgery, prolonged operating time, or operational failure. Therefore, accurate localization of the target lesion before resection is crucial for surgical planning to achieve positive oncological outcomes while preserving residual lung function and reducing the operating time.
Rationale and knowledge gap
With more limited surgical access, localization of lung lesions becomes even more challenging, as small nodules are impalpable and invisible to the naked eye. Previous cohorts had suggested localization when the target was less than 10 mm in size, with more than 5 mm distance to the nearest pleural surface (9,11). An expert consensus recently suggested localization in small lung nodules <15 mm, deep-seated nodules >10 mm from the visceral pleura, and pure GGN or semi-solid nodules (12). Ideally, a perfect localization technique should have high accuracy, low procedure-related complications, minimize patient discomfort, and be cost-effective. Nowadays, various localization methods have been developed to overcome this challenge, including new imaging modalities and localization marker placement. With more innovative technologies, such as robotic-assisted bronchoscopy (RAB), tumor-specific markers and augmented reality (AR) now being incorporated into the localization process to improve accuracy and minimize complications, these technologies are under ongoing investigation in order to examine the potential clinical advantages. This is an updated review on the latest evidence of these novel localization techniques, especially on the use of RAB and electromagnetic navigational bronchoscopy (ENB) for lung nodule localization, of which review on latest evidence is still lacking.
Objective
The objective of this review is to examine various localization techniques currently used in MIS and VATS, and to understand their advantages and limitations in different situations. Furthermore, we reviewed the possible future directions of the latest technology.
Methods
We identified data by searching PubMed from 1990 to 2023 and from references cited in relevant articles. The following search terms were used: lung/pulmonary nodule, localization, hook wire, micro coil, fiducial marker, indocyanine green (ICG), methylene blue (MB), lipiodol, contrast, radiotracer, fluorescence marker, intraoperative lung ultrasound, electromagnetic navigation bronchoscopy, computed tomography (CT) reconstruction, manual/finger palpation, AR, hybrid operating room/theater, tumor-specific marker, and tissue-specific marker. Only case reports written in English were included. The abstracts were excluded from this study.
Advantages and disadvantages of each localization technique were summarized in Table 1.
Table 1
Techniques | Advantages | Disadvantages |
---|---|---|
Manual palpation | (I) No extra equipment required; (II) low cost; (III) minimal trauma to lung parenchyma/low risk profile |
(I) Highly operator dependent; (II) low accuracy in subsolid nodule; (III) technically challenging in MIS |
ILU | Minimal trauma to lung parenchyma/low risk profile | (I) Operator dependent; (II) require complete lung deflation; (III) air-trapping artefact; (IV) lower accuracy in subsolid nodule |
3D CT reconstruction | (I) Non-invasive; (II) no extra operative risk | (I) Require specialized software and regular calibration of software; (II) discrepancy between inflated and deflated lungs leading to inaccurate localization |
ENB | (I) Allow co-localization; (II) high success rate; (III) lower risk of pneumothorax |
Lack of “bronchus sign” leads to lower success rate |
RAB | (I) Allow co-localization; (II) high success rate; (III) lower risk of pneumothorax; (IV) better ergonomics and instrument stability | Lack of “bronchus sign” leads to lower success rate |
ICG | (I) Satisfactory success rate; (II) short procedural time; (III) cheap | (I) Risk of pneumothorax (in percutaneous injection); (II) risk of parenchymal diffusion/contamination; (III) lower success rate in deep-seated lesion; (IV) false-positive in inflammatory lesion (in intravenous ICG); (V) required specialized camera |
MB | (I) Visualized by naked vision; (II) short procedural time; (III) satisfactory success rate; (IV) cheap | (I) Risk of pneumothorax (in percutaneous injection); (II) risk of parenchymal diffusion/contamination; (III) lower success rate in deep-seated lesion; (IV) anaphylactic reaction; (V) poorly identified in anthracotic lungs; (VI) affect histopathological exam of surgical specimen |
Contrast agents | (I) Longer retaining time; (II) can be used with other liquid agents | (I) Can cause inflammatory reaction; (II) risk of embolism; (III) risk of pneumothorax (in percutaneous injection); (IV) require intra-operative fluoroscopy |
Radiotracers | (I) Isotopes have long retaining time; (II) satisfactory success rate | (I) Need high specialized equipment; (II) radiation exposure; (III) risk of pneumothorax (in percutaneous injection) |
Hookwire | (I) Short procedural time; (II) cheap; (III) satisfactory success rate; (IV) visible to naked vision | (I) Risk of wire dislodgement; (II) higher risk of pneumothorax; (III) not suitable for certain anatomical locations; (IV) patient discomfort |
Microcoil/gold fiducial marker/magnetic-guided occult lesion localization | (I) Short procedural time; (II) satisfactory success rate | (I) Require fluoroscopy; (II) risk of marker migration/marker embolization; (III) risk of pneumothorax (in percutaneous placement) |
HOR | (I) Shorter procedural time; (II) one-stop solution, minimize delay between localization and lung resection; (III) allow co-localization | High cost |
AR | (I) Allow virtual guidance; (II) non-invasive | Relatively high cost |
Tumor-specific marker | (I) Tumor-specific; (II) higher localization rate in deep-seated nodule; (III) might be able to identify additional malignancy lesions | May cause adverse drug reaction |
RFID | Visualized by both fluoroscopy and CT | (I) Need additional RFID detector; (II) risk of pneumothorax (in percutaneous placement) |
ILU, intra-operative lung ultrasonography; CT, computed tomography; ENB, electromagnetic navigational bronchoscopy; RAB, robotic-assisted bronchoscopy; ICG, indocyanine green; MB, methylene blue; HOR, hybrid operating room; AR, augmented reality; RFID, radiofrequency identification; MIS, minimally invasive surgery.
Manual palpation
Manual palpation is the oldest method used in both conventional thoracotomy and MIS. It involves no extra equipment but relies heavily on the surgeon’s tactile sense for the lung nodule localization within the normal lung parenchyma. Hence, manual palpation is often the first-line technique for nodule localization in solid lung metastasectomy, such as in osteosarcoma metastases, because the solid consistency of osteosarcoma metastases makes manual palpation easier. In a systematic review of 1,472 patients who underwent open thoracotomy for lung metastasectomies, manual palpation was shown to have a high sensitivity with a 100% success rate in detecting solid lung metastases. Although this systematic review reported a false-positive rate of 13–48.5% when using manual palpation (13), it is interesting to note that manual palpation could potentially reveal undiagnosed lung metastases at the time of surgery. Parsons et al. retrospectively reviewed 34 patients who underwent lung metastasectomy and reported 22% more malignant nodules detected intraoperatively with manual palpation than those detected by preoperative CT (14); this indicates that manual palpation during pulmonary metastasectomy could alter intraoperative decision-making and improve clinical outcomes. However, due to the heavy reliance on the difference in density between normal lung parenchyma and abnormal lung nodules, smaller nodules in deeper locations and subsolid nodules make manual palpation in thoracoscopic surgery less sensitive (15). Sensitivity to the detection of semi-solid nodules is reported to be only approximately 30% (9). The effectiveness of this technique also depends on surgical access. As one might expect, a smaller wound in MIS makes manual palpation challenging since the surgeon’s hand is limited by both access and reach to the whole lung for complete assessment. Therefore, manual palpation is rarely used as the sole technique in lung nodule localization, especially in small and subsolid nodules, and is usually used in conjunction with other modalities, such as intraoperative ultrasound.
Imaging modalities
Intra-operative lung ultrasonography (ILU) has been used for intraoperative lung nodule localization since the 1990s. It utilizes an ultrasonic probe with a 10 mm linear tip and is a real-time and non-invasive tool that does not damage the lung parenchyma and has a very low-risk profile. During use, the lung must be fully deflated to avoid artifacts caused by the air trapped within. Furthermore, the probe should be placed on the pleural surface of the area of interest. Detection of lung nodules is based on the difference in density, which is displayed as different echogenicity between normal lung parenchyma and lung nodules on ultrasonography. Solid lung nodules appear as a hyperechoic lesion with a hypoechoic shadow. In GGN, the density is similar to that of the adjacent lung parenchyma, which might make ultrasonic detection difficult. However, Kondo et al. reported a cohort of 44 patients with a 100% GGN detection rate, with ILU performed by experienced operators, of which 62% were pure GGN (16). In a prospective observational cohort, ILU demonstrated a sensitivity of 93% in detecting lung nodules versus a sensitivity of 40% using palpation alone. The positive predictive value of ILU was 100% (17). Taurchini et al. demonstrated a 100% sensitivity for detecting small lung nodules <2 cm in size and centrally located lung nodules in 131 patients (18). No complications were reported in any of the cohorts mentioned above. Although ILU is a safe and non-invasive tool for detecting lung nodules with different solidarity and size, it is highly operator-dependent. Furthermore, it has limited accuracy in patients with emphysematous lung disease or patients with incomplete lung deflation during surgery, such as those who cannot tolerate one-lung ventilation.
Technology for CT imaging is continuously advancing. In recent decades, thin-cut CT has been reconstructed into 3D models using specialized software programs such as the Materialize Interactive Medical Image Control System (MIMICS), Belgium. This software can create a 3D representation of the lungs, showing details of each lobe, pleural and fissure contours, and surrounding bronchovascular structures. The target lung nodule can be displayed within the model, and the operator can determine the position of the nodule in relation to different anatomical landmarks. Efficient surgical planning can be performed to achieve a precise anatomical lung resection using this 3D model; this facilitates surgeons in performing sublobar resection or segmentectomy in patients with small and subsolid nodules (19). It is beneficial in multifocal lung tumors, where surgical planning with the 3D model allows multiple sublobar resections for complete tumor resection while preserving the residual lung volume (20). Nodule localization using the reconstructed 3D model is feasible with a low-risk profile, as it is non-invasive and causes no additional discomfort. Zhang et al. reported satisfactory outcomes when using visual localization with 3D CT reconstruction and proved that it could be applied to small, subsolid, and deeply located lung nodules. Of 121 lung nodules, 117 were localized successfully using 3D CT reconstruction; 91.7% were semi-solid or pure GGN, the mean nodule diameter was 7.67 mm, and the mean distance from visceral pleura was 14.84 mm (20). It is worth noting that this technique has some limitations. First, it requires specialized software that must be purchased and updated regularly by the developer. Second, the lungs were inflated on the preoperative CT but deflated during the actual operation, accounting for the slight discrepancy in the precise location of the nodule compared to the modeled one. This technique is commonly used with intraoperative manual palpation and mapping programs such as virtual bronchoscopy.
Conventional flexible bronchoscopy has limited use in lung nodule localization because of its inability to reach the distal airways. In recent years, ENB has rapidly become a vital tool for managing small lung nodules, including diagnosis (21,22), localization (23), and even treatment (24,25). ENB utilizes specialized software with electromagnetic positionings, such as the SuperDimension Navigation System by Medtronic, USA. This specialized program can analyze a preoperative CT and format the set of CT images into a 3D roadmap of the bronchial tree. The target lung nodule is displayed on the roadmap, and a virtual pathway is created via which the bronchoscope can be precisely guided toward the target nodule. Through the instrument channel of the navigation bronchoscope, various tools can be used to perform localization, including injecting different liquid agents, such as dye marking (23) and placement of metallic fiducial markers (26). Potential benefits of ENB localization include a reduced risk of pneumothorax, shorter procedural time, minimal delay between localization and lung resection, the ability to localize multiple lung nodules in a single procedure, and the flexibility of performing biopsy at the same time (27,28). Previous studies have demonstrated a high success rate with a low-risk profile. Abbas et al. reported a 98.1% success rate using ENB localization in 51 patients with both solid nodules and GGN. No cases of operative mortality were reported (29). A propensity-matched study by Kuo et al. demonstrated no significant difference in the localization success rate between ENB-guided and CT-guided dye marking. Both localization methods achieved high success rates of 93.3% and 90% in the ENB and CT groups, respectively. However, they reported that the ENB group had a significantly shorter total procedural time, less delay between localization and surgery, and fewer cases of pneumothorax (6.7% vs. 36.7%) (30). Kuo’s findings were consistent with a previous cohort study by Bolton et al., in which a 100% success rate was reported with the use of ENB localization. Compared to CT-guided localization, a significantly shorter total procedure and waiting time between localization and resection (27 vs. 189 min) have been reported (27). Although ENB showed promising results for lung nodule localization, a ‘bronchus sign’ on pre-procedural CT was noted to indicate successful navigation. In a prospective single-center study including 51 patients, 79% had a ‘bronchus sign’, while only 31% were diagnosed with nodules without a ‘bronchus sign’. In both univariate and multivariate analysis, the presence of ‘bronchus sign’ was statistically significant in successful diagnosis (31).
RAB has been introduced to automate navigation bronchoscopy further and improve accuracy and ergonomics. In addition to navigational systems, the bronchoscope is held by a robotic articulating arm, while the surgeon controls the robotic arm remotely with a remote control (Figure 1A,1B). With a steady robotic arm, the surgeon can advance the bronchoscope through narrower and more distal bronchioles, which would otherwise be difficult to reach. More complex localization procedures can also be achieved because of the exact spatial orientation (32). In a multicenter prospective study conducted by Chen et al., 54 patients underwent lung nodule biopsy using a RAB with electromagnetic navigation. Once the robotic scope was positioned at the targeted nodule, radial endobronchial ultrasound was used to confirm the lesion localization. They reported successful localization in 96.2% of the patients using RAB and a low rate of adverse events; pneumothorax occurrence was 3.7%, and no major airway injury was observed (33). Chaddha et al. reported similar results in their retrospective review of 165 patients who underwent RAB for peripheral lung nodule biopsy. The successful navigation rate was 88.6%, with pneumothorax occurring in 3.6% of patients and airway bleeding in 2.4% (34). With these encouraging results, we can foresee RAB becoming a game-changer for peripheral lung nodule localization and diagnosis. Ongoing clinical trials, such as BENEFIT and TARGET, will provide more evidence for using RAB.
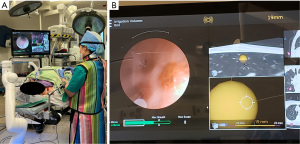
Marker localization
ICG is a fluorescent contrast liquid agent that emits or absorbs 820 nm fluorescence and becomes visible under a near-infrared (NIR) camera. It is a universal liquid agent used in localization techniques because of its availability and relatively deep tissue penetration (35). There are three main methods of ICG administration for lung nodule localization, which are also applicable to other liquid agents.
First, ICG can be used for CT-guided percutaneous localization. The procedure was performed under local anesthesia immediately before lung resection, using CT to localize the target nodule and injecting a small volume of ICG near the target nodule under CT guidance. Intraoperatively, a NIR thoracoscope was used to detect the fluorescence (Figure 2). CT-guided ICG localization has a high success rate and a low false-negative rate. A meta-analysis by Gkikas et al. reported an overall localization success rate of 97.6% and a low false-negative rate of 2.4% (36). Li et al. retrospectively reviewed patients who underwent CT-guided ICG localization and reported a localization success rate of 98.4% (37). Another advantage of using percutaneous ICG localization is the short procedure time; the reported duration of localization time ranged from 6 to 59 min in the meta-analysis (36), while Li et al. reported an average procedure time of 18 min (37). The usual complications reported were pneumothorax (5.9%), intra-parenchymal hematoma (6.8%), and mild hemoptysis (0.4%) (37). Most of these complications are related to lung puncture rather than the reaction to ICG.
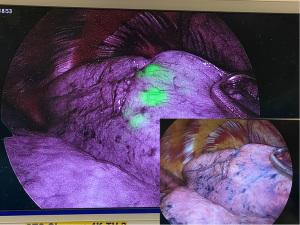
Furthermore, percutaneous localization in deep-seated nodules has a potentially increased risk of pneumothorax because of the long distance traveled by the puncture needle. ICG fluorescence diminishes as the location deepens and diffuses into the surrounding lung parenchyma, reducing its accuracy in localizing deep-seated nodules. A recent expert consensus recommended the application of image-guided or bronchoscopic ICG localization for lesions located no deeper than 2 cm from pleural surface (38). A technique using a mixture of emulsifying agents, commonly Lipiodol, and ICG to perform localization was developed. Rho et al. reported a 100% success rate for deep-seated nodules with a mean depth of 12 mm from the pleura using this mixture (39). This technique is limited by the depth of the lung nodule and the select nodule location, such as those close to the mediastinal structures and major vasculature.
Similarly, ICG can be injected via a bronchoscopic approach, which carries a lower rate of complications than CT-guided percutaneous ICG injection due to the lack of visceral pleural puncture (40). This method, as opposed to the percutaneous approach, is more favorable for lung nodules that are centrally located and close to the bronchial tree. A meta-analysis showed an overall success rate of 95.4% using bronchoscopic ICG localization, and the reported procedure duration ranged from 3 to 45 min (36). A prospective study comparing CT-guided percutaneous injection versus bronchoscopic ICG injection for lung nodule localization showed a 100% success rate in the percutaneous group versus 93.8% in the bronchoscopic group. The risk of pneumothorax was 20% in the percutaneous group, and none was observed in the bronchoscopic group. Hence, it was proposed that the bronchoscopic approach is suitable for marking multiple lung nodules using a single procedure (41).
Finally, intravenous (IV) ICG injection for localization is currently being studied. Theoretically, porous tumor blood vessels have higher permeability, resulting in the accumulation of ICG when administered intravenously. ICG is administered intravenously around 12–24 hours before the operation, allowing sufficient time for ICG to be retained within the lung nodule. In a prospective study including 11 patients who received 1 mg/kg intravenous ICG injected 24 h before lung resection, the localization success rate was 90.9%, and the false positive rate was 18.2%. Hence, one of the limitations of IV ICG localization is that passive accumulation of ICG cannot accurately distinguish lung neoplasms from inflammation (42). Okusanya et al. performed IV ICG localization in 18 patients in a pilot study and found that 88.9% of the nodules were successfully localized. Five additional subcentimeter nodules were localized, including three metastatic adenocarcinomas and two metastatic sarcomas (43). No severe allergic reactions were reported in any of the cohorts. Hence, the expert consensus in 2022 acknowledged the safety and feasibility of intravenous ICG in lung nodule localization. However, they also acknowledged the possibility of false positive result due to detection of inflammatory lesions (38).
MB is a widely used liquid dye marker for lung nodule localization. Unlike ICG, MB can be visualized as an intense dark blue stain under normal light. Therefore, specialized cameras are not required during operation. Like ICG, MB can be injected near a target lung nodule via the CT-guided percutaneous or endobronchial approach. Shentu et al. reported a successful localization rate of 94% and a mean procedure time of 17.4 min (44). Complications reported in recent cohorts include pneumothorax and pulmonary hemorrhage. The risk of pneumothorax was high in patients with multiple nodules, and the risk of pulmonary hemorrhage was positively correlated with nodule depth (45). Although rare, anaphylactic reactions to MB have been reported in the literature. The major limitations of MB localization are that it diffuses rapidly into the surrounding lung parenchyma and may not be visible when injected at a distance from the pleura. It has also been poorly identified in severe anthracosis lungs (40). Due to the diffusion tendency, the time interval between MB injection and lung resection must be short to minimize MB diffusion into the surrounding tissue, leading to failed localization.
Contrast agents, such as Lipiodol and Barium, are less regularly used as the only marker in lung nodule localization. They are water-insoluble media that can be injected via CT-guided percutaneous injection or bronchoscopic-guided endobronchial injection. Barium is the less-used medium, leading to an acute inflammatory reaction and edema of the surrounding lung tissue. These reactions may have been falsely interpreted as lung nodules. On the other hand, Lipiodol is usually used with another liquid agent, such as ICG, to improve the localization of deep-seated lung nodules and reduce diffusion (39). The main advantage of water-insoluble agents is their ability to be retained in a small area of the lung parenchyma for a more extended period and the reduced need for immediate lung resection after injection. In a study by Watanabe et al., a high success localization rate of 100% was achieved using CT-guided Lipiodol localization. Complications reported included pneumothorax (17%), chest pain (11%), hemoptysis (6%), and hemopneumothorax (0.6%) (46). Lipiodol poses the risk of embolism because of its water insolubility. Similar to all contrast agents, Lipiodol requires real-time fluoroscopic localization. Therefore, both patients and medical personnel are exposed to increased radiation.
Radiotracers were first reported in 2000 (47). Radioguided occult lesion localization (ROLL) is comparatively less utilized because it requires highly specialized facilities, including radiation protection equipment, specialized radiologists, and gamma probes. Compared to other techniques, it is less cost-effective. Radioisotopes such as Technetium-99 are attached to large albumin molecules. This combined molecule emits gamma rays, which can then be detected by a handheld gamma probe used intraoperatively. Furthermore, optional scintigraphy can be performed before lung resection to confirm the presence of radiotracers. Since radioisotopes can remain stable for 24 hours, there is no rush to perform lung resection immediately after injection of the radiotracer. A previous study by Ambrogi et al. reported a 99% success rate in 211 patients with a mean target nodule size of 8.3 mm. There were no severe complications, except that 10% of patients developed a small pneumothorax (48). Similarly, Starnes et al. reported a primary success rate of 92.4%, with 9% of patients developing pneumothorax and one developing hemothorax (49).
A randomized controlled trial compared radiotracer and hookwire localization and demonstrated a success rate of 96% with radiotracer localization in contrast to 84% with hookwire localization. The difference in success rates was accounted for by localization failure caused by wire dislodgement. Twenty-four percent of patients in the hookwire group developed pneumothorax, whereas 4% of patients in the radiotracer group developed a pneumothorax (50). Radiotracers must be removed entirely from the patient’s body because of their radioactivity.
Among all localization techniques, fiducial markers such as hookwire and microcoil are among the most used and studied, with image-guided percutaneous hookwire localization, first described in the 1990s, as the prime example (51). Hookwire localization can be performed under fluoroscopic guidance or, more commonly, under CT guidance. With the patient in either the supine or prone position, lung nodules were located on CT, and the most straightforward pathway from the skin to the target nodule was planned. Under local anesthesia and CT guidance, a needle introducer with a hookwire was inserted percutaneously into the lung while carefully advancing the needle introducer until it reached the target nodule. The hookwire is then released and anchored into the lung nodule, leaving a semi-rigid wire protruding through the pleura and emerging from the skin. Immediately after hook wire placement, CT or fluoroscopy was used to confirm the position and to identify possible complications, such as pneumothorax and pulmonary hemorrhage. Hookwire localization has a satisfactory success rate and short localization time, but it has certain significant drawbacks regarding dislodgement and anatomical limitations (52). Zhang et al. reported a successful localization rate of 100% in patients with small lung nodules (mean diameter of 8.5 mm). Hookwire dislodgement was observed in 2.5% of patients. This retrospective cohort’s mean procedural time was nine minutes (53). Another cohort study reported by Hanauer et al. showed a comparable result; they reported a 100% localization rate in 181 patients. However, seven patients (3.7%) had hookwire dislodgement, and lung resection was made possible by identifying the visceral pleura puncture site (54). Complications reported were consistent between different cohorts (50-53), with 33.7–40% of asymptomatic pneumothorax, 0–2.1% of symptomatic pneumothorax, and 5.9–36% of bleeding complications (54). Hookwire dislodgement commonly occurs in three situations: (I) during transportation to the operation theatre; (II) at the beginning of the operation when the lung has to be deflated; and (III) during surgical manipulation. The maneuvering and transportation of patients can be limited using a hybrid operating room (HOR) to minimize the risk of dislodgement. Seo et al. investigated the factors affecting successful CT-guided hookwire localization in a retrospective cohort of 174 patients. The localization success rate was 95%, and wire dislodgement was found in all eight failure cases (4.95%). On multivariate analysis, they identified that the distance between the wire tip and pleural surface was the only independent factor for successful hookwire localization (55). Realistically, lung nodules that are deeply located in the lung parenchyma, shielded by the scapula, or close to the mediastinum/hilar are technically challenging to locate; this increases the risk of injury to bronchovascular structures and, therefore, limits the application of hookwire localization.
Previous studies comparing MB and ICG against hookwire localization showed no statistically significant difference in localization success rate. However, hookwire localization is associated with a higher occurrence of complications. Kleedehn et al. compared the results between the hookwire and MB groups and demonstrated an equivalent localization rate and a wire dislodgement rate of 13%. The risks of pneumothorax and pulmonary hemorrhage were higher in the hookwire group (38% vs. 25% and 12% vs. 4%, respectively) (56). Ding et al. compared the results of CT-guided ICG and hookwire localization. The localization rate was 100% in the ICG group and 95.6% in the hookwire group, explained by the occurrence of dislodgement of the hookwire. Overall complications and mean pain scores were higher in the hookwire group (57).
To aid hookwire localization, another innovative localization method that utilizes 3D printing technology has also been reported. In a pilot study of 16 patients, individualized navigational models were created by processing preoperative CT images using computer-aided design software. The models were then exported to a 3D printer to produce the physical navigational templates. The physical templates were placed on the thoracic wall and used as a guide for the CT-guided hookwire localization. The success rate was 100% using this template-guided localization in the pilot study (58). Subsequently, the same investigators reported their results from the randomized clinical trial with 190 patients, comparing 3D template-guided versus CT-guided hookwire localization. They showed that the efficacy and safety of template-guided hookwire localization are non-inferior to those of CT-guided hookwire localization, and template-guided localization significantly reduced patient radiation exposure, from the mean exposure of 313 mGy·cm in CT-guided group to 229 mGy·cm in template-guided group (59).
Alternative types of fiducial markers, namely platinum microcoils and gold fiducial markers, have been investigated to solve this dislodgement problem. The implantation procedure was similar to that used for the hookwire localization. Under CT or fluoroscopy guidance, a needle introducer containing a microcoil is passed into the patient’s body and guided toward the target nodule. Once the position was confirmed, the coil was deployed, and its distal end was embedded entirely into the lung parenchyma. The needle introducer was removed, leaving the superficial end at the visceral pleura. No wire protruded extracorporeally (60). Since no part of the wire was left behind, the patient’s discomfort and the risk of dislodgement were reduced. In a randomized controlled trial comparing patients with microcoil localization with patients not receiving preoperative localization, microcoil localization was shown to increase the successful diagnosis rate and shorten the operative time (61). Compared to hookwire, metallic fiducial markers cannot easily be visualized and require fluoroscopic guidance during surgery to locate the marker. Hence, in addition to the usual complications of pneumothorax and pulmonary hemorrhage, the disadvantages of using metallic fiducial include additional radiation exposure for medical personnel, marker migration, and rarely, marker embolization. McGuire et al. reported their 5-year study at a single institution; the successful localization rate in 92 patients was 100%. However, they also reported frequent complications, although most were minor and did not require any treatment. These included pneumothorax (46.4%), lung hematoma (18.6%), coil dislodgement (4.1%) and hemoptysis (2.1%) (62). In a recent retrospective cohort study, Teng et al. reported a 98.5% localization rate in 31 patients with multiple lung nodules and a 12.9% pneumothorax rate (63). Thus, microcoil localization is an efficient method with an acceptable risk profile.
Newer technique of magnetic-guided occult lesion localization (M-GOLL) utilizing magnetic fiducial marker has been reported by Garcia and colleagues. The deployment technique of M-GOLL is similar to those used in conventional fiducial marker localization. After the placement of magnetic marker, a magnetic sensing probe is used intra-operatively to detect the exact location of the marker by providing both audio and proximity-based numeric feedback. In their cohort of 12 patients, 100% success localization rate was achieved. Three patients developed pneumothorax (25%) and one of them required chest tube drainage for pneumothorax (8.3%) (64).
Use of HOR
The time interval between lung nodule localization and lung resection affects the localization accuracy in the above-mentioned techniques. For example, a prolonged duration between CT-guided percutaneous dye marking and VATS leads to excessive dye diffusion. In contrast, a prolonged duration between CT-guided percutaneous hook wire localization and VATS leads to an increased risk of wire dislodgement or worsened discomfort. The use of HOR has been advocated to address this concern. The HOR is a magnetic and radiation-compatible operating theatre equipped with CBCT and fluoroscopy; this provides a one-stop solution for lung nodule management and smoothens the workflow between lung nodule localization and VATS (Figure 3). This streamlined workflow avoids delays between localization and surgery and has been proven to reduce the risk of hookwire dislodgement and pneumothorax (65,66). More importantly, patient discomfort can be minimized with fewer delays between the localization and VATS. Using the HOR, multiple localization techniques (co-localization) can be performed in a single setting. Triple dye injection with fiducial markers into the deep margin is commonly performed. In particular, the triple dye included an equal volume of iohexol contrast, MB, and ICG solution. In HOR, the triple dye is injected into the target nodule, and, optionally, fiducial markers can be placed into the deep margin via ENB or RAB (Figure 4A,4B). The dye and fiducial positions can be confirmed intraoperatively by CBCT. The patient can then be positioned and prepared for VATS. During VATS, the MB can be visualized under a normal white light thoracoscope, while ICG can be visualized after switching to a NIR camera. Using real-time fluoroscopy in HOR, contrast and fiducial markers can be visualized. When used in combination, these techniques can improve localization accuracy and ensure the inclusion of deep margins (23,26). Leow et al. reported the result of lung nodule localization in HOR among 159 patients with 174 lung nodules. They performed nodule localization using a range of techniques, including both CT-guided transthoracic and ENB-guided bronchoscopic placement of marker or dye. Single- or double-marker technique was utilized. The overall localization success rate was 95.9%, which failed localization was due to pneumothorax (1.7%), microcoil dislodgement (0.57%) and machine failure (1.7%). Overall rate of pneumothorax occurrence was 10.7% (67). Yu et al. reviewed their experience with image-guided VATS using the HOR in 32 patients. Image-guided hookwire localization in the HOR was performed by a radiologist using CBCT. Subsequently, the patient was immediately repositioned and underwent VATS. On-table fluoroscopy or CBCT can be used to confirm the position of the hookwire. A 100% nodule localization was achieved, and pneumothorax was detected in 47% of patients; however, none of the patient required intervention, as they all underwent VATS immediately. No hookwire dislodgement was observed in this cohort, and there was much less delay between localization and surgery (41.1 min) than between standard hook wire localization and VATS (109.5 min) (66). A recent cohort study published by Gilberto et al. also reported a 100% localization rate in HOR using hookwire, microcoil, or dye marking techniques. Of the 20 patients, two developed pneumothorax that required drainage, and one had microcoil displacement (65). The above studies demonstrate that localization in the HOR is an effective and safe approach. It allows co-localization with various real-time imaging modalities and reduces the delay between localization and surgery. Thus, the potential risks of wire dislodgement and pneumothorax are minimized.
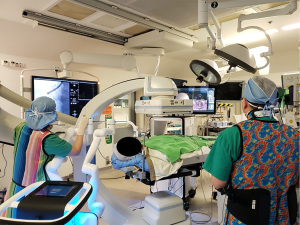
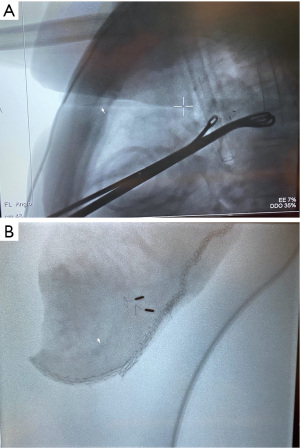
Heading into the future—upcoming techniques
Augmented reality
In the near future, we can expect continuous development of ENB and RAB in managing small lung nodules. AR is currently on the horizon for improving the precision of small lung nodule localization. AR uses computer-generated images and projects them onto a real-life environment as an overlay. An AR headset is an apparatus that allows the operator to wear a 3D model of the patient’s anatomy and the target lung nodule to be displayed virtually in the operator’s surgical field. Surgical planning and localization pathways can be determined and executed exactly by following the virtual pathway displayed in the operator’s headset view. One such AR headset on the market is the HoloLens. Currently, no human trial has been conducted, but AR headsets have been studied in animal models. Li et al. published a study using canine models of artificial peripheral pulmonary lesions. Pre-procedural CT was performed on the animals, and the CT images were analyzed using a special program with a localization plan developed. The images and localization plan were uploaded to the HoloLens. Localization markers were implanted into canine models under HoloLens guidance. All 12 artificial lesions were successfully localized, and the average distance between the lesions and markers was 1.9 mm. No severe pneumothorax was observed (68). However, this futuristic technique is undergoing perpetual investigation to determine its real-world applications in different clinical settings, such as the accuracy of image projection during lung inflation and deflation.
Tumor-specific marker
Ideally, a tumor-specific dye should be used when performing lung nodule localization to minimize staining of the normal lung parenchyma and to reduce background interference. Although intravenous ICG has been investigated and has shown a high success rate for lung nodule localization, ICG is not tumor-specific and can be detected in non-malignant lung nodules. Furthermore, spillage and rapid diffusion of ICG are occasionally observed, affecting localization accuracy (36). An experimental tumor-specific dye, OTL-38 (CYTALUX™), was developed to address this problem. Folate receptor is highly expressed in approximately 80% of lung adenocarcinomas. On the other hand, OTL-38 is a tumor-specific molecule that binds to folate receptors and is linked to a fluorophore which can be detected under a NIR camera. In a human trial, preoperative intravenous injection of OTL-38 combined with an intraoperative NIR camera allowed the detection of 15 out of 21 lung lesions, which was superior to traditional localization with manual finger palpation and direct visualization under white-light VATS, which detected only 10 out of 21 lung lesions. It was also interesting to observe that relatively deep-seated lesions up to 2–2.7 cm in depth could be successfully localized using OTL-38. No toxicity was observed in this study (69). Another recent multicenter phase two trial studied the use of OTL-38 and its influence on intraoperative decision-making. With OTL-38, additional malignant lesions were identified intraoperatively, which would otherwise have been missed. Detecting additional lesions altered surgical management and improved outcomes in 26% of the patients. Similarly, no significant toxicity or complications were reported in this study, although mild adverse drug effects, mainly nausea, were observed (70). The results using OTL-38 are encouraging; however, more evidence is required to determine its usefulness in localizing pre-malignant lesions. The U.S. Food and Drug Administration (FDA) has approved the use of OTL-38 (CYTALUX™) in identifying cancerous lung nodule. A phase 3 randomized clinical trial is in progress, aims at studying the safety and efficacy of OTL-38 injection in localizing folate-receptor positive lung nodules.
Radiofrequency identification (RFID)
Among the use of physical markers, Sato and colleagues introduced the first application of RFID device into lung nodule localization in 2020 (71). RFID tag is a small metallic marker that can communicate with a hand-held antenna via radiofrequency. RFID tag is implanted as a physical marker via percutaneous or bronchoscopic technique. During VATS, a hand-held detector is used to detect radiofrequency signal and to locate the lung nodule. A retrospective cohort including 39 patients underwent RFID localization and subsequently sublobar resection performed, complete resection with clear margin was achieved in all patients. Mean distance between tag and lung nodule was 2.9 mm (72). This new device offers alternative for fiducial marker placement, not only it is visualized on fluoroscopy or CT. Radiofrequency emitted by the tag can be detected by detector intra-operatively for additional accuracy.
The major limitation of this literature review is the non-systematic nature and the potential selection bias as the study selection was performed by one reviewer alone. Nonetheless, this review included a wide range of clinical studies and covered the latest evidence on various localization techniques. It provides a comprehensive overview on the topic and sheds light on the future direction of developing techniques.
Conclusions
With an increase in the use of CT screening, the prevalence of small and subsolid lung nodules is also exponentially increasing. Various localization techniques have been developed to address the challenges in localizing these small lung nodules. However, there is no consensus on the choice of technique as it depends on the operator’s expertise, resource availability, and nodule characteristics. The true paradigm shift in this era is the application of co-localization with the use of HOR, which allows the operator to combine multiple imaging modalities and localization techniques in a one-stop manner. New technologies such as AR are undergoing extensive investigation and with them being incorporated into daily practice, we are getting closer to a perfect localization technique with high accuracy and low morbidities.
Acknowledgments
Funding: None.
Footnote
Peer Review File: Available at https://vats.amegroups.com/article/view/10.21037/vats-22-64/prf
Conflicts of Interest: All authors have completed the ICMJE uniform disclosure form (available at https://vats.amegroups.com/article/view/10.21037/vats-22-64/coif). CSHN serves as an unpaid editorial board member of Video-Assisted Thoracic Surgery from August 2021 to July 2025. RWHL is a consultant for Medtronic, USA and Siemens Healthineer, Germany. CSHN is a consultant for Johnson and Johnson, USA, Medtronic, USA and Siemens Healthineer, Germany. The other authors have no conflicts of interest to declare.
Ethical Statement: The authors are accountable for all aspects of the work in ensuring that questions related to the accuracy or integrity of any part of the work are appropriately investigated and resolved.
Open Access Statement: This is an Open Access article distributed in accordance with the Creative Commons Attribution-NonCommercial-NoDerivs 4.0 International License (CC BY-NC-ND 4.0), which permits the non-commercial replication and distribution of the article with the strict proviso that no changes or edits are made and the original work is properly cited (including links to both the formal publication through the relevant DOI and the license). See: https://creativecommons.org/licenses/by-nc-nd/4.0/.
References
- Thandra KC, Barsouk A, Saginala K, et al. Epidemiology of lung cancer. Contemp Oncol (Pozn) 2021;25:45-52. [Crossref] [PubMed]
- Jonas DE, Reuland DS, Reddy SM, et al. Screening for lung cancer with low-dose computed tomography. JAMA 2021;325:971. [Crossref] [PubMed]
- Gould MK, Donington J, Lynch WR, et al. Evaluation of individuals with pulmonary nodules: When is it lung cancer? Chest 2013;143:e93S-e120S. [Crossref] [PubMed]
- Ng CSH, MacDonald JK, Gilbert S, et al. Optimal Approach to Lobectomy for Non-Small Cell Lung Cancer: Systemic Review and Meta-Analysis. Innovations (Phila) 2019;14:90-116. [Crossref] [PubMed]
- Yim AP, Izzat MB, Liu HP, et al. Thoracoscopic major lung resections: an Asian perspective. Semin Thorac Cardiovasc Surg 1998;10:326-31. [Crossref] [PubMed]
- Yim AP, Wan S, Lee TW, et al. VATS lobectomy reduces cytokine responses compared with conventional surgery. Ann Thorac Surg 2000;70:243-7. [Crossref] [PubMed]
- Li WW, Lee RL, Lee TW, et al. The impact of thoracic surgical access on early shoulder function: video-assisted thoracic surgery versus posterolateral thoracotomy. Eur J Cardiothorac Surg 2003;23:390-6. [Crossref] [PubMed]
- Ng CS, Lee TW, Wan S, et al. Thoracotomy is associated with significantly more profound suppression in lymphocytes and natural killer cells than video-assisted thoracic surgery following major lung resections for cancer. J Invest Surg 2005;18:81-8. [Crossref] [PubMed]
- Suzuki K, Nagai K, Yoshida J, et al. Video-assisted thoracoscopic surgery for small indeterminate pulmonary nodules: indications for preoperative marking. Chest 1999;115:563-8. [Crossref] [PubMed]
- Saito H, Minamiya Y, Matsuzaki I, et al. Indication for preoperative localization of small peripheral pulmonary nodules in thoracoscopic surgery. J Thorac Cardiovasc Surg 2002;124:1198-202. [Crossref] [PubMed]
- Kim MP, Nguyen DT, Chan EY, et al. Computed tomography criteria for the use of advanced localization techniques in minimally invasive thoracoscopic lung resection. J Thorac Dis 2018;10:3390-8. [Crossref] [PubMed]
- Liu B, Gu C. Expert consensus workshop report: Guidelines for preoperative assisted localization of small pulmonary nodules. J Cancer Res Ther 2020;16:967-73. [Crossref] [PubMed]
- Macherey S, Doerr F, Heldwein M, et al. Is manual palpation of the lung necessary in patients undergoing pulmonary metastasectomy? Interact Cardiovasc Thorac Surg 2016;22:351-9. [Crossref] [PubMed]
- Parsons AM, Detterbeck FC, Parker LA. Accuracy of helical CT in the detection of pulmonary metastases: is intraoperative palpation still necessary? Ann Thorac Surg 2004;78:1910-6; discussion 1916-8. [Crossref] [PubMed]
- Ichinose J, Mun M, Matsuura Y, et al. Efficiency of thoracoscopic palpation in localizing small pulmonary nodules. Surg Today 2019;49:921-6. [Crossref] [PubMed]
- Kondo R, Yoshida K, Hamanaka K, et al. Intraoperative ultrasonographic localization of pulmonary ground-glass opacities. J Thorac Cardiovasc Surg 2009;138:837-42. [Crossref] [PubMed]
- Khereba M, Ferraro P, Duranceau A, et al. Thoracoscopic localization of intraparenchymal pulmonary nodules using direct intracavitary thoracoscopic ultrasonography prevents conversion of VATS procedures to thoracotomy in selected patients. J Thorac Cardiovasc Surg 2012;144:1160-5. [Crossref] [PubMed]
- Taurchini M, Quarato CMI, Frongillo EM, et al. Intraoperative Lung Ultrasound (ILU) for the Assessment of Pulmonary Nodules. Diagnostics (Basel) 2021;11:1691. [Crossref] [PubMed]
- Kanzaki M, Kikkawa T, Shimizu T, et al. Presurgical planning using a three-dimensional pulmonary model of the actual anatomy of patient with primary lung cancer. Thorac Cardiovasc Surg 2013;61:144-50. [Crossref] [PubMed]
- Zhang G, Xu D, Yu Z, et al. Preoperative non-invasive visual localization of synchronous multiple lung cancers using three-dimensional computed tomography lung reconstruction. J Cardiothorac Surg 2021;16:273. [Crossref] [PubMed]
- Folch EE, Pritchett MA, Nead MA, et al. Electromagnetic Navigation Bronchoscopy for Peripheral Pulmonary Lesions: One-Year Results of the Prospective, Multicenter NAVIGATE Study. J Thorac Oncol 2019;14:445-58. [Crossref] [PubMed]
- Jiang S, Xie F, Mao X, et al. The value of navigation bronchoscopy in the diagnosis of peripheral pulmonary lesions: A meta-analysis. Thorac Cancer 2020;11:1191-201. [Crossref] [PubMed]
- Ng CSH, Zhao Z, Long H, et al. Electromagnetic Navigation Bronchoscopy Triple Contrast Dye Marking for Lung Nodule Localization. Thorac Cardiovasc Surg 2020;68:253-5. [Crossref] [PubMed]
- Zhao ZR, Lau RWH, Ng CSH. Catheter-based alternative treatment for early-stage lung cancer with a high-risk for morbidity. J Thorac Dis 2018;10:S1864-70. [Crossref] [PubMed]
- Chan JWY, Lau RWH, Ngai JCL, et al. Transbronchial microwave ablation of lung nodules with electromagnetic navigation bronchoscopy guidance-a novel technique and initial experience with 30 cases. Transl Lung Cancer Res 2021;10:1608-22. [Crossref] [PubMed]
- Chan JWY, Lau RWH, Ng CSH. Electromagnetic navigation bronchoscopy fiducial marker margin identification plus triple dye for complete lung nodule resection. JTCVS Tech 2020;3:329-33. [Crossref] [PubMed]
- Bolton WD, Cochran T, Ben-Or S, et al. Electromagnetic Navigational Bronchoscopy Reduces the Time Required for Localization and Resection of Lung Nodules. Innovations (Phila) 2017;12:333-7. [Crossref] [PubMed]
- Mariolo AV, Vieira T, Stern JB, et al. Electromagnetic navigation bronchoscopy localization of lung nodules for thoracoscopic resection. J Thorac Dis 2021;13:4371-7. [Crossref] [PubMed]
- Abbas A, Kadakia S, Ambur V, et al. Intraoperative electromagnetic navigational bronchoscopic localization of small, deep, or subsolid pulmonary nodules. J Thorac Cardiovasc Surg 2017;153:1581-90. [Crossref] [PubMed]
- Kuo SW, Tseng YF, Dai KY, et al. Electromagnetic Navigation Bronchoscopy Localization Versus Percutaneous CT-Guided Localization for Lung Resection via Video-Assisted Thoracoscopic Surgery: A Propensity-Matched Study. J Clin Med 2019;8:379. [Crossref] [PubMed]
- Seijo LM, de Torres JP, Lozano MD, et al. Diagnostic yield of electromagnetic navigation bronchoscopy is highly dependent on the presence of a Bronchus sign on CT imaging: results from a prospective study. Chest 2010;138:1316-21. [Crossref] [PubMed]
- Agrawal A, Hogarth DK, Murgu S. Robotic bronchoscopy for pulmonary lesions: a review of existing technologies and clinical data. J Thorac Dis 2020;12:3279-86. [Crossref] [PubMed]
- Chen AC, Pastis NJ Jr, Mahajan AK, et al. Robotic Bronchoscopy for Peripheral Pulmonary Lesions: A Multicenter Pilot and Feasibility Study (BENEFIT). Chest 2021;159:845-52. [Crossref] [PubMed]
- Chaddha U, Kovacs SP, Manley C, et al. Robot-assisted bronchoscopy for pulmonary lesion diagnosis: results from the initial multicenter experience. BMC Pulm Med 2019;19:243. [Crossref] [PubMed]
- Ujiie H, Kato T, Hu HP, et al. A novel minimally invasive near-infrared thoracoscopic localization technique of small pulmonary nodules: A phase I feasibility trial. J Thorac Cardiovasc Surg 2017;154:702-11. [Crossref] [PubMed]
- Gkikas A, Lampridis S, Patrini D, et al. How effective is indocyanine green (ICG) in localization of malignant pulmonary nodules? A systematic review and meta-analysis. Front Surg 2022;9:967897. [Crossref] [PubMed]
- Li X, Xu K, Cen R, et al. Preoperative computer tomography-guided indocyanine green injection is associated with successful localization of small pulmonary nodules. Transl Lung Cancer Res 2021;10:2229-36. [Crossref] [PubMed]
- Ng CS, Ong BH, Chao YK, et al. Use of Indocyanine Green Fluorescence Imaging in Thoracic and Esophageal Surgery. Ann Thorac Surg 2023;115:1068-76. [Crossref] [PubMed]
- Rho J, Lee JW, Quan YH, et al. Fluorescent and Iodized Emulsion for Preoperative Localization of Pulmonary Nodules. Ann Surg 2021;273:989-96. [Crossref] [PubMed]
- Tajè R, Gallina FT, Forcella D, et al. Fluorescence-guided lung nodule identification during minimally invasive lung resections. Front Surg 2022;9:943829. [Crossref] [PubMed]
- Anayama T, Hirohashi K, Miyazaki R, et al. Near-infrared dye marking for thoracoscopic resection of small-sized pulmonary nodules: comparison of percutaneous and bronchoscopic injection techniques. J Cardiothorac Surg 2018;13:5. [Crossref] [PubMed]
- Kim HK, Quan YH, Choi BH, et al. Intraoperative pulmonary neoplasm identification using near-infrared fluorescence imaging. Eur J Cardiothorac Surg 2016;49:1497-502. [Crossref] [PubMed]
- Okusanya OT, Holt D, Heitjan D, et al. Intraoperative near-infrared imaging can identify pulmonary nodules. Ann Thorac Surg 2014;98:1223-30. [Crossref] [PubMed]
- Shentu Y, Zhang L, Gu H, et al. A new technique combining virtual simulation and methylene blue staining for the localization of small peripheral pulmonary lesions. BMC Cancer 2014;14:79. [Crossref] [PubMed]
- Lin CY, Chang CC, Huang LT, et al. Computed Tomography-Guided Methylene Blue Localization: Single vs. Multiple Lung Nodules. Front Med (Lausanne) 2021;8:661956. [Crossref] [PubMed]
- Watanabe K, Nomori H, Ohtsuka T, et al. Usefulness and complications of computed tomography-guided lipiodol marking for fluoroscopy-assisted thoracoscopic resection of small pulmonary nodules: experience with 174 nodules. J Thorac Cardiovasc Surg 2006;132:320-4. [Crossref] [PubMed]
- Chella A, Lucchi M, Ambrogi MC, et al. A pilot study of the role of TC-99 radionuclide in localization of pulmonary nodular lesions for thoracoscopic resection. Eur J Cardiothorac Surg 2000;18:17-21. [Crossref] [PubMed]
- Ambrogi MC, Melfi F, Zirafa C, et al. Radio-guided thoracoscopic surgery (RGTS) of small pulmonary nodules. Surg Endosc 2012;26:914-9. [Crossref] [PubMed]
- Starnes SL, Wolujewicz M, Guitron J, et al. Radiotracer localization of nonpalpable pulmonary nodules: A single-center experience. J Thorac Cardiovasc Surg 2018;156:1986-92. [Crossref] [PubMed]
- Gonfiotti A, Davini F, Vaggelli L, et al. Thoracoscopic localization techniques for patients with solitary pulmonary nodule: hookwire versus radio-guided surgery. Eur J Cardiothorac Surg 2007;32:843-7. [Crossref] [PubMed]
- Mack MJ, Gordon MJ, Postma TW, et al. Percutaneous localization of pulmonary nodules for thoracoscopic lung resection. Ann Thorac Surg 1992;53:1123-4. [Crossref] [PubMed]
- Ichinose J, Kohno T, Fujimori S, et al. Efficacy and complications of computed tomography-guided hook wire localization. Ann Thorac Surg 2013;96:1203-8. [Crossref] [PubMed]
- Zhang H, Li Y, Yimin N, et al. CT-guided hook-wire localization of malignant pulmonary nodules for video assisted thoracoscopic surgery. J Cardiothorac Surg 2020;15:307. [Crossref] [PubMed]
- Hanauer M, Perentes JY, Krueger T, et al. Pre-operative localization of solitary pulmonary nodules with computed tomography-guided hook wire: report of 181 patients. J Cardiothorac Surg 2016;11:5. [Crossref] [PubMed]
- Seo JM, Lee HY, Kim HK, et al. Factors determining successful computed tomography-guided localization of lung nodules. J Thorac Cardiovasc Surg 2012;143:809-14. [Crossref] [PubMed]
- Kleedehn M, Kim DH, Lee FT, et al. Preoperative pulmonary nodule localization: a comparison of methylene blue and hookwire techniques. AJR Am J Roentgenol 2016;207:1334-9. [Crossref] [PubMed]
- Ding N, Wang K, Cao J, et al. Targeted near-infrared fluorescence imaging with iodized indocyanine green in preoperative pulmonary localization: comparative efficacy, safety, patient perception with Hook-Wire localization. Front Oncol 2021;11:707425. [Crossref] [PubMed]
- Zhang L, Li M, Li Z, et al. Three-dimensional printing of navigational template in localization of pulmonary nodule: A pilot study. J Thorac Cardiovasc Surg 2017;154:2113-2119.e7. [Crossref] [PubMed]
- Zhang L, Wang L, Kadeer X, et al. Accuracy of a 3-dimensionally printed navigational template for localizing small pulmonary nodules: a noninferiority randomized clinical trial. JAMA Surg 2019;154:295-303. [Crossref] [PubMed]
- Mayo JR, Clifton JC, Powell TI, et al. Lung nodules: CT-guided placement of microcoils to direct video-assisted thoracoscopic surgical resection. Radiology 2009;250:576-85. [Crossref] [PubMed]
- Finley RJ, Mayo JR, Grant K, et al. Preoperative computed tomography-guided microcoil localization of small peripheral pulmonary nodules: a prospective randomized controlled trial. J Thorac Cardiovasc Surg 2015;149:26-31. [Crossref] [PubMed]
- McGuire AL, Vieira A, Grant K, et al. Computed tomography-guided platinum microcoil lung surgery: A cross-sectional study. J Thorac Cardiovasc Surg 2019;158:594-600. [Crossref] [PubMed]
- Teng F, Wu AL, Yang S, et al. Preoperative computed tomography-guided coil localization for multiple lung nodules. Ther Adv Respir Dis 2020;14:1753466620909762. [Crossref] [PubMed]
- González García JA, Rodríguez Alvarado EI, Gutiérrez Ramírez MC, et al. Magnetic-guided occult lesion localization (M-GOLL): an exploratory feasibility study of preoperative CT-guided puncture and intraoperative magnetic seed localization for minimally invasive surgical resection of non-palpable pulmonary lesions. Ann Surg Oncol 2023;30:1309-11. [Crossref] [PubMed]
- Gilberto GM, Falsarella PM, Andrade JR, et al. Lung nodule localization in hybrid room before minimally invasive thoracic surgery: series of 20 cases and literature review. Einstein (Sao Paulo) 2022;20:eAO6665. [Crossref] [PubMed]
- Yu PSY, Man Chu C, Lau RWH, et al. Video-assisted thoracic surgery for tiny pulmonary nodules with real-time image guidance in the hybrid theatre: the initial experience. J Thorac Dis 2018;10:2933-9. [Crossref] [PubMed]
- Leow OQY, Chao YK. Individualized strategies for intraoperative localization of non-palpable pulmonary nodules in a hybrid operating room. Front Surg 2019;6:32. [Crossref] [PubMed]
- Li C, Zheng Y, Yuan Y, et al. Augmented reality navigation-guided pulmonary nodule localization in a canine model. Transl Lung Cancer Res 2021;10:4152-60. [Crossref] [PubMed]
- Predina JD, Newton A, Corbett C, et al. Localization of pulmonary ground-glass opacities with folate receptor-targeted intraoperative molecular imaging. J Thorac Oncol 2018;13:1028-36. [Crossref] [PubMed]
- Gangadharan S, Sarkaria IN, Rice D, et al. Multiinstitutional phase 2 clinical trial of intraoperative molecular imaging of lung cancer. Ann Thorac Surg 2021;112:1150-9. [Crossref] [PubMed]
- Sato T, Yutaka Y, Nakamura T, et al. First clinical application of radiofrequency identification (RFID) marking system-precise localization of a small lung nodule. JTCVS Tech 2020;4:301-4. [Crossref] [PubMed]
- Ueda Y, Mitsumata S, Matsunaga H, et al. Use of a radiofrequency identification system for precise sublobar resection of small lung cancers. Surg Endosc 2023;37:2388-94. [Crossref] [PubMed]
Cite this article as: Chang ATC, Chan JWY, Siu ICH, Yu PSY, Lim K, Wan IYP, Lau RWH, Ng CSH. Lung nodule localization techniques in minimally invasive and video-assisted thoracoscopic surgery: a literature review. Video-assist Thorac Surg 2023;8:29.