Resident education in robotic thoracic surgery
Introduction
For nearly two decades, the integration and clinical use of robotics has undergone continual growth and evolution. The Food and Drug Administration (FDA) approved the first robot-assisted platform in 2000, and as of March 2019, there are 5,114 da Vinci Surgical Systems (Intuitive Surgical Inc., Sunnyvale, CA, USA) in use. The first reported case of robotic lobectomy came in 2002 (1) and since that time robotic thoracic procedures have expanded in numbers and complexity. Many experienced surgeons already adept at open or video-assisted thoracoscopic surgery (VATS) have incorporated robotics into their practice. A review of The Society of Thoracic Surgeons (STS) database from 2006 to 2016 showed 271 thoracic surgeons had performed a total of 5,619 robotic lobectomies (2).
Robotic surgery offers several important advantages including: (I) three-dimensional view; (II) enhanced magnification; (III) improved precision and degrees of movement; (IV) surgeon-controlled retraction and camera platform; eliminating variability in assistant skill level; (V) surgeon ergonomics and comfort; (VI) ease of hilar and mediastinal lymph node dissection. Disadvantages include the cost of acquisition (approximately $1.8 million) with an additional 10% annual service fee and instrument cost ($700–1,000 per case) (3). Lack of haptic feedback has also been cited as a disadvantage; however, it has not been shown to correlate with poorer outcomes.
Early studies comparing robot-assisted procedures to traditional minimally invasive techniques consistently demonstrated safety and equivalent outcomes, however robotics was associated with increased expense and prolonged operative times (4-7). This led many to argue that robotics simply resulted in increased cost while yielding similar results. However, with more widespread adoption, advancements in technology, and new market competition it is foreseeable that the cost of robotics will continue to come down and advantages will become more apparent.
Despite the popularity and growth in robotic thoracic procedures, there is no standardized training curriculum and a paucity of research exists in regards to the optimal training paradigm. In a survey of recent cardiothoracic graduates, the majority of respondents reported they lacked confidence in robotic pulmonary operations (55.8%) and robotic esophageal operations (61.5%) (8). The aim of this review is to discuss the increasing role of robotics in thoracic surgery, examine the literature and evidence in other surgical specialties regarding optimal training and education, and describe essential elements necessary for the successful implementation of a thoracic surgery robotic training program.
Robotic surgery education and training
Robotics has been adopted by numerous specialties including gynecology, urology, general surgery, colorectal surgery, and cardiothoracic surgery. It is estimated that over 1.5 million robotic surgeries have already been performed (9). Currently, general surgery represents the fastest growing field in robotics (10,11). A national survey of general surgery residents reports 96% of residents have a robotic platform where they train, however only 18% reported operating at the console (12). A recent study of general surgery program directors found a need for increased emphasis on robotic surgery and training, and a more standardized path to competency. Most programs (80%) relied on industry to assist in resident education, as cost and simulation access was a noted to be a major obstacle (13).
Training in laparoscopy includes the Fundamentals of Laparoscopic Surgery (FLS) training curriculum, which tests both cognitive and technical skills and is a requirement for certification as a general surgeon. While similar programs such as Fundamental Skills of Robot-Assisted Surgery (FSRS) and Fundamentals of Robotic Surgery (FRS) have been developed, they have not been similarly implemented or mandated (13,14). Some have argued the integration of robotics has significant educational and financial opportunity cost to trainees which should be considered. In one report, robotics led to an upward shift in the level of residents performing operations, and a reduction in overall resident participation (11). This potential pitfall should be addressed when building and incorporating robotics into any training program.
Training paradigms in other specialties have been well described (15-17). Although not-standardized, they generally consist of three distinct training phases: First, a preclinical phase which includes online didactics, and skill acquisition with simulation models. Second, is the bedside assistant phase. Trainees learn port placement, docking, instrument exchange, and troubleshooting. Lastly, trainees progress to the console phase and participate in parts of the robotic operation in a progressive fashion. In general surgery training, phase one typically occurs during PGY1, phase two as a PGY2–3, and phase 3 during the PGY4–5 years (13). There is a growing sentiment, however, to push for earlier exposure and progression through each phase.
Current paradigms in robotic thoracic training
Adopting new technology is a challenge in any training program. There is currently no standardized robotic curriculum or case requirement for thoracic surgery trainees (18). However, several institutions have reported their experience and strategy in regards to building a robotic curriculum (19-21). The goal of these programs is to provide a balanced experience so that trainees become proficient in open, VATS, and robotic thoracic techniques.
The University of Alabama has reported extensively on their robotic experience and educational approach (22-25). In a series of 520 consecutive robotic lobectomies, authors demonstrate resident involvement increased over time and did not negatively impact patient outcomes (26). While operative time initially was longer, that tended to decrease overtime with experience. Resident involvement was tracked by breaking each lobectomy down into a series of steps, and each step was allotted a set amount of time (for example, residents were given 7 min to remove lymph nodes at stations 9, 8, and 7) (26). This enabled accurate data collection, and acted as a measure to track individual trainee progression.
Other institutions have reported their results following implementation of robotics into their thoracic surgery curriculum. The University of Michigan reported results following their first 100 robotic cases (38% lung resections, 23% esophageal operations, and 20% sympathectomies). Resident involvement increased in the later cohort (33% to 59%). Operative time and postoperative complications were similar regardless of the level of resident participation (9).
The variability in resident experience and prior training in robotics is an important consideration which is unique to thoracic surgery training programs. There are currently several pathways in cardiothoracic surgery including traditional fellowship following completion of general surgery residency, integrated I-6, and 4+3 programs (27). This adds additional complexity when designing an educational curriculum, as it must be flexible and tailored to the individual trainee.
Thoracic robotic program—essential elements
While standardization within residency training programs is lacking, there is a clear pathway for resident and fellows to obtain da Vinci certification (Table 1). Ideally this would be obtained during general surgery training and prior to thoracic surgery fellowship. However, for fellows with limited robotic exposure or for integrated residents within a thoracic surgery training program, this should be pursued as early as possible. This training essentially follows previously established modular curriculums which include pre-clinical, bedside assistant, and console phases, and can be used to frame a resident curriculum.
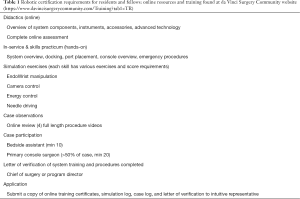
Full table
Following successful completion of a training program, trainees should possess the skills and certifications necessary for hospital credentialing. Although these requirements vary widely based on the institution, a rigorous training program will provide graduates with an easy pathway (28). Identification of key elements for a thoracic surgery training program (Table 2) were derived from literature review and the authors’ own institutional experience.
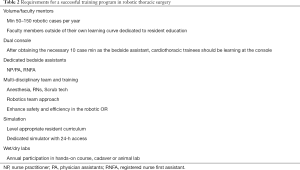
Full table
Faculty mentors
Teaching is a skill, and the best teachers are master surgeons who possess the passion and dedication to training the next generation of surgeons. A successful robotic training program should have robotic surgeons who are beyond their own learning curve in order to be effective resident educators. For those still learning, a classification system for thoracic operations and a pathway to competency in robotic thoracic surgery has been proposed (25). Many surgeons who are adept at teaching VATS may struggle to provide equivalent autonomy during robotics until they gain sufficient experience and comfort. While the number varies, the learning curve for an already proficient VATS surgeon is approximately 20 robotic lobectomies (29,30). Annual volume is also important to offer a robust training experience, a minimum volume of 50–150 robotic cases per year is required.
To succeed, faculty mentors also require institutional support. This includes dedicated robotic operative time each week and recognition that teaching is an investment. In today’s atmosphere there is pressure to limit operative time, drive down cost, and increase volume all while outcomes are under heightened scrutiny. These factors have been recognized as reasons faculty members have become “disincentivized” to teach (31).
Robotic procedures can easily be recorded and reviewed at a later date. Video-based coaching has been shown to aid in skill development and operative technique (32). Faculty mentors should review surgical videos and provide feedback to residents as well as their bedside assistants. This review process can also inform the mentor by providing valuable feedback on coaching style and allow for reflection on the quantity and quality of teaching they are providing (31).
Dual console
Training residents using a single-console system has several important disadvantages. The observer monitor is two dimensional and the trainee is required to switch seats with the mentor to resume control of the console. This requires time, changes to console ergonomics, and forces the mentor and trainee to be physically apart. A dual-console system (Figure 1) enables the trainee and mentor to have the same three-dimensional view, easily swap controls, or operate simultaneously. Simultaneous operation, while more advanced, can give the trainee control of three arms while the mentor controls a retracting arm for example.
Mentors can also utilize on-screen pointers, and resume control without changing sides of the table as in traditional surgery. Studies on dual-console experience in gynecology and urology have demonstrated increased collaboration between trainee and mentor, decreased operative times, and decreased intraoperative and postoperative complications (33-35).
Dedicated bedside assistants
Robotic surgeons, while in close proximity are physically away from the bedside, and therefore must place trust in the bedside assistant. The impact of an experienced bedside assistant cannot be understated. They maintain consistency, help orient unfamiliar operating room staff, ensure proper positioning, port placement, docking, instrument exchange, and in many cases perform critical tasks throughout the case via an assistant port.
In many academic centers, surgical residents with varying levels of experience function as the “bedside surgeon.” Some have suggested this has led to a decline in operative autonomy, case volume, and concluded that implementation of robotics into a training program comes at a “cost (11).” It is our belief that permanent highly trained and experienced bedside assistants are critical for any robotic thoracic surgery program (Figure 2). They help maintain consistency, safety, and allow residents to train on the console. In the United States, a variety of advanced practice providers (APPs) such as physician assistants (PAs), or registered nurses (RNs) or nurse practitioners (NPs) who have trained as registered nurse first assistants (RNFAs). In Europe, surgical care practitioners (SCPs) have similar training and analogous function. New bedside assistants should complete the same online didactics and hands-on training sessions required for residents and fellows (Table 1).
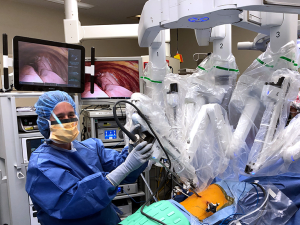
Robotics team
Surgery is a team sport, and that sentiment is perhaps most evident in robotic thoracic surgery. It is essential that a multi-disciplinary approach be taken to training and preparation. Having a dedicated team of anesthesiologists, surgeons, bedside assistants, nurses, and technicians is instrumental to the success of a thoracic surgery robotic training program. Training a smaller number of team members establishes familiarity and helps improve efficiency. Nurses and technicians must master several perioperative competencies: arranging the robotic system and all its components in the OR, assembling system components, starting the system, draping the patient cart, setting up and calibrating the vision system, setting up the surgeon console, driving and positioning the patient cart over the patient, identifying safety features, and performing system shutdown (36).
Robotic surgery poses unique challenges to effective communication. There are physical, visual, and auditory barriers unique to robotic surgery, and traditional nonverbal communication tools cannot be deployed (37). To ensure proper flow of information, instructions from the console surgeon should be clear, and the bedside assistant should repeat those instructions back for confirmation. Reduction in ambient noise, as well as adjuncts to the da Vinci audio system such as the use of headsets, may be needed to improve intraoperative team communication.
Previous reports have shown that having an established robotic team can decrease the learning curve for individual surgeons (38). This team is a necessary component for a robotic thoracic training program in order to teach good practices and maintain an optimal learning environment for trainees.
Simulation
The development and expansion of simulation-based education is taking place in nearly every surgical specialty. The ability to reduce adverse and life-threatening events has been demonstrated in multiple fields including avionics and medicine. With increasing demands to improve patient safety, simulation is playing a larger role in skills acquisition. Simulation is devoid of risk to the patient, and shortening the learning curve prior to patient contact has the goal of improved operative experience and outcomes. Various forms of simulation exist and include: robotic table top models (Figure 3), virtual reality (VR) surgical simulators, live animal labs, and cadaveric training. With simulation, thoracic trainees can gain familiarity with the robotic platform as well as learn anatomy and surgical approach to thoracic procedures (e.g., lobectomy, mediastinal dissection).
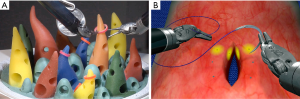
Like laparoscopy, many robotic skills can first be learned in a laboratory. However, unlike laparoscopy which has numerous inexpensive platforms and even take-home mobile box-trainers (39), robotic simulators come at a substantial cost. The initial acquisition cost of a da Vinci skills simulator (dVSS) is approximately $85,000 with additional annual maintenance fees (40). Importantly, the dVSS cannot function independently and requires a da Vinci Surgical system console. In comparison, the estimated cost of establishing a laparoscopic simulation lab is $7,706 with an annual maintenance cost of $5,036 (41).
VR simulation ranges from basic skills with performance analysis to procedural based simulation (e.g., lobectomy simulation). Computer based VR simulation has the added advantage of measurable competence-based tasks. These metrics can be tracked and used to ensure a basic level of competence and exposure prior to resident involvement in robotic cases. There are currently a handful of commercially available platforms in addition to the da Vinci Skills Simulator. These include the robotic surgical simulator (RoSS; Simulated Surgical Systems LLC, San Jose, CA, USA), dV-Trainer (Mimic Technologies, Seattle, WA, USA), and the SEP Robot (SimSurgery, Oslo, Norway). These other stand-alone simulation platforms have been shown to offer similar attributes at a comparable cost (range $40,000–100,000) (40,42).
Simulation has now become an essential component for any robotic thoracic training program. To be the most effective, simulation must be available and easily accessible to surgical trainees who are often inundated with demands of patient care and training. Ideally, simulators should be housed in a simulation center with 24-hour access and have a vision monitor that allows a user or tutor to observe and provide feedback. For many programs this is cost prohibitive and a major limitation. Some institutions have made the investment by making a single simulator available across departments as a shared resource.
Hands-on training labs
In order to maintain proficiency and get exposure to new technology annual attendance of some form hands-on robotics training is recommended. These can include industry or institution sponsored cadaveric or animal labs, or implementation of mock dry runs and use of table top models. On-site training is also available through SAGES Robotics Masters Series, FSRS training program, and the robotics training network (RTN) curriculum (43).
The American Association for Thoracic Surgery (AATS) offers a surgical robotics fellowship providing advanced educational experiences for thoracic trainees and attendings surgeons in a two-day course. Advanced robotic lobectomy courses are also available to fellows who meet the case requirements. In addition, Intuitive offers a variety of mentorship, proctoring, and observation opportunities for fellows and faculty.
Future directions
Currently, robotic surgery generates nearly $3 billion in annual revenue and that number is projected to climb. Intuitive Surgical has led the field with innovative technology and advanced platforms such as their newest robot, the da Vinci Xi. Aggressive marketing and various forms of sponsorship have also likely contributed to the popularization of robotics for surgeons, hospitals, and patients. Given the financial incentives involved, it is imperative that unbiased research be conducted to evaluate these novel technologies, and authors must declare any financial conflicts of interest to improve transparency (44).
Intuitive has now developed the da Vinci SP, a single-port access robotic using a single arm to manipulate 3 arms and a camera, and in February of 2019, they received FDA clearance for the Ion Endoluminal system. The Ion is a flexible robotic-assisted catheter system for gaining access to distal airways. Advancements in these systems have exciting implications for robotic thoracic surgery, creating both challenge and opportunity for surgeons in training and the programs tasked with integrating innovation into their curriculum.
Given the growth in popularity and potential revenue it is not surprising that robotic competitors are emerging. Unique robotic platforms have been developed by companies such as TransEnterix, Titan Medical, Human Xtensions, and Medrobotics (45). The Senhance system (TransEnterix Surgical Inc. Morrisville, NC, USA) received FDA clearance in 2017 and has been in use in Europe and now the US. This system features a console with an eye-tracking feature, providing the possibility of moving the camera with eye movement, and incorporates haptic feedback. Importantly, to reduce cost this platform also has reusable instruments and is designed to work with commercially available trocars (46).
In addition to already established competitive platforms, there are several others under development. VERB Surgical is a robotic collaboration between Google and Johnson and Johnson. Their stated goal is to advance digital surgery claiming, “surgical technology is about to take a giant leap forward.” However, no detailed information has been released to date. Finally, it is anticipated that Medtronics, in collaboration with Karl Storz SE &Co., will introduce their robotic platform within the year. Like VERB surgical, little is known in terms of specifics but many anticipate compatibility with existing laparoscopic towers and instruments (45,47).
As more systems become available perhaps one of the largest drawbacks to robotics will be addressed – the cost. Reducing upfront acquisition and per case cost will ensure robotics continues to expand. New challenges will need to be confronted as robotic training, credentialing, and outcomes research will become inherently more complex with multiple platforms being introduced.
Conclusions
As the role of robotics in thoracic surgery continues to evolve, educators must maintain their proficiency and deliver training utilizing the newest minimally invasive techniques. Thoracic surgery training programs should include a robust experience in robotic surgery, and to maximize success should incorporate the key elements outlined in this article. As with any new technology, priority is first to provide optimal patient outcomes with oncologically sound operations. However, there is little doubt that robotic platforms will continue to improve and become an increasingly important skill set in the thoracic surgeons’ repertoire.
Acknowledgments
Funding: None.
Footnote
Provenance and Peer Review: This article was commissioned by the Guest Editors (Natalie S. Lui and Sean C. Wightman) for the series “Robotic Surgery for Lung Cancer” published in Video-Assisted Thoracic Surgery. The article has undergone external peer review.
Conflicts of Interest: Both authors have completed the ICMJE uniform disclosure form (available at http://dx.doi.org/10.21037/vats.2020.01.01). The series “Robotic Surgery for Lung Cancer” was commissioned by the editorial office without any funding or sponsorship. NSL served as the unpaid Guest Editor of the series. NSL reports non-financial support from Intuitive Surgical, outside the submitted work. The authors have no other conflicts of interest to declare.
Ethical Statement: The authors are accountable for all aspects of the work in ensuring that questions related to the accuracy or integrity of any part of the work are appropriately investigated and resolved.
Open Access Statement: This is an Open Access article distributed in accordance with the Creative Commons Attribution-NonCommercial-NoDerivs 4.0 International License (CC BY-NC-ND 4.0), which permits the non-commercial replication and distribution of the article with the strict proviso that no changes or edits are made and the original work is properly cited (including links to both the formal publication through the relevant DOI and the license). See: https://creativecommons.org/licenses/by-nc-nd/4.0/.
References
- Melfi FM, Menconi GF, Mariani AM, et al. Early experience with robotic technology for thoracoscopic surgery. Eur J Cardiothorac Surg 2002;21:864-8. [Crossref] [PubMed]
- Feczko AF, Wang H, Nishimura K, et al. Proficiency of Robotic Lobectomy Based on Prior Surgical Technique in the STS General Thoracic Database. Ann Thorac Surg 2019;108:1013-20. [Crossref] [PubMed]
- Schreuder HW, Wolswijk R, Zweemer RP, et al. Training and learning robotic surgery, time for a more structured approach: a systematic review. BJOG 2012;119:137-49. [Crossref] [PubMed]
- Breitenstein S, Nocito A, Puhan M, et al. Robotic-assisted versus laparoscopic cholecystectomy: outcome and cost analyses of a case-matched control study. Ann Surg 2008;247:987-93. [Crossref] [PubMed]
- Melvin WS, Needleman BJ, Krause KR, et al. Computer-enhanced vs. standard laparoscopic antireflux surgery. J Gastrointest Surg 2002;6:11-5. [Crossref] [PubMed]
- Delaney CP, Lynch AC, Senagore AJ, et al. Comparison of robotically performed and traditional laparoscopic colorectal surgery. Diseases of the colon & rectum 2003;46:1633-9. [Crossref] [PubMed]
- Swanson SJ, Miller DL, McKenna RJ Jr, et al. Comparing robot-assisted thoracic surgical lobectomy with conventional video-assisted thoracic surgical lobectomy and wedge resection: results from a multihospital database (Premier). J Thorac Cardiovasc Surg 2014;147:929-37. [Crossref] [PubMed]
- Chu D, Vaporciyan AA, Iannettoni MD, et al. Are there gaps in current thoracic surgery residency training programs? Ann Thorac Surg 2016;101:2350-5. [Crossref] [PubMed]
- White YN, Dedhia P, Bergeron EJ, et al. Resident training in a new robotic thoracic surgery program. J Surg Res 2016;201:219-25. [Crossref] [PubMed]
- Ahmad A, Ahmad ZF, Carleton JD, et al. Robotic surgery: current perceptions and the clinical evidence. Surg Endosc 2017;31:255-63. [Crossref] [PubMed]
- Mehaffey JH, Michaels AD, Mullen MG, et al. Adoption of robotics in a general surgery residency program: at what cost? J Surg Res 2017;213:269-73. [Crossref] [PubMed]
- Farivar BS, Flannagan M, Leitman IM. General surgery residents’ perception of robot-assisted procedures during surgical training. J Surg Educ 2015;72:235-42. [Crossref] [PubMed]
- George LC, O’Neill R, Merchant AM. Residency training in robotic general surgery: a survey of program directors. Minim Invasive Surg 2018;2018:8464298 [Crossref] [PubMed]
- Smith R, Patel V, Satava R. Fundamentals of robotic surgery: a course of basic robotic surgery skills based upon a 14‐society consensus template of outcomes measures and curriculum development. Int J Med Robot 2014;10:379-84. [Crossref] [PubMed]
- Gómez Ruiz M, Alfieri S, Becker T, et al. Expert consensus on a train‐the‐trainer curriculum for robotic colorectal surgery. Colorectal Dis 2019;21:903-8. [Crossref] [PubMed]
- Winder JS, Juza RM, Sasaki J, et al. Implementing a robotics curriculum at an academic general surgery training program: our initial experience. J Robot Surg 2016;10:209-13. [Crossref] [PubMed]
- Moles JJ, Connelly PE, Sarti EE, et al. Establishing a training program for residents in robotic surgery. Laryngoscope 2009;119:1927-31. [Crossref] [PubMed]
- Veronesi G, Dorn P, Dunning J, et al. Outcomes from the Delphi process of the Thoracic Robotic Curriculum Development Committee. Eur J Cardiothorac Surg 2018;53:1173-9. [Crossref] [PubMed]
- Terra RM, Haddad R, de Campos JRM, et al. Building a Large Robotic Thoracic Surgery Program in an Emerging Country: Experience in Brazil. World J Surg 2019;43:2920-6. [Crossref] [PubMed]
- Herrera L, DaSilva M. Training program in robotic thoracic surgery. J Vis Surg 2018;4:1. [Crossref] [PubMed]
- Shahin GM, Bruinsma GJBB, Stamenkovic S, et al. Training in robotic thoracic surgery—the European way. Ann Cardiothorac Surg 2019;8:202. [Crossref] [PubMed]
- Linsky PL, Wei B. Training in robotic thoracic surgery. J Vis Surg 2018;4:1. [Crossref] [PubMed]
- Cerfolio RJ, Bryant AS, Minnich DJ. Starting a robotic program in general thoracic surgery: why, how, and lessons learned. Ann Thorac Surg 2011;91:1729-36; discussion 1736-7.
- Wahl TS, Wei B. Surgical simulation in robotic-assisted thoracic surgery: training. Video-assist Thorac Surg 2018;3:46. [Crossref]
- Cerfolio RJ, Bryant AS. How to teach robotic pulmonary resection. Semin Thorac Cardiovasc Surg 2013;25:76-82. [Crossref] [PubMed]
- Cerfolio RJ, Cichos KH, Wei B, et al. Robotic lobectomy can be taught while maintaining quality patient outcomes. J Thorac Cardiovasc Surg 2016;152:991-7. [Crossref] [PubMed]
- Wahl TS, Wei B. Certification for minimally invasive thoracic surgery: is your surgeon ready? J Thorac Dis 2019;11:S1145. [Crossref] [PubMed]
- Bhora FY, Al-Ayoubi AM, Rehmani SS, et al. Robotically assisted thoracic surgery: proposed guidelines for privileging and credentialing. Innovations 2016;11:386-9. [Crossref] [PubMed]
- Veronesi G. Robotic lobectomy and segmentectomy for lung cancer: results and operating technique. J Thorac Dis 2015;7:S122. [PubMed]
- Melfi FM, Mussi A. Robotically assisted lobectomy: learning curve and complications. Thorac Surg Clin 2008;18:289-95. [Crossref] [PubMed]
- Cerfolio RJ, Ferrari-Light D. How to get the most out of your trainees in robotic thoracic surgery—“the coachability languages Ann Cardiothorac Surg 2019;8:269. [Crossref] [PubMed]
- Hu YY, Peyre SE, Arriaga AF, et al. Postgame analysis: using video-based coaching for continuous professional development. J Am Coll Surg 2012;214:115-24. [Crossref] [PubMed]
- Morgan MS, Shakir NA, Garcia-Gil M, et al. Single-versus dual-console robot-assisted radical prostatectomy: impact on intraoperative and postoperative outcomes in a teaching institution. World J Urol 2015;33:781-6. [Crossref] [PubMed]
- Hanly EJ, Miller BE, Kumar R, et al. Mentoring console improves collaboration and teaching in surgical robotics. J Laparoendosc Adv Surg Tech A 2006;16:445-51. [Crossref] [PubMed]
- Smith AL, Krivak TC, Scott EM, et al. Dual-console robotic surgery compared to laparoscopic surgery with respect to surgical outcomes in a gynecologic oncology fellowship program. Gynecol Oncol 2012;126:432-6. [Crossref] [PubMed]
- Zender J, Thell C. Developing a successful robotic surgery program in a rural hospital. AORN J 2010;92:72-83; quiz 84-6. [Crossref] [PubMed]
- Schiff L, Tsafrir Z, Aoun J, et al. Quality of communication in robotic surgery and surgical outcomes. JSLS 2016; [Crossref] [PubMed]
- Sim HG, Yip SKH, Lau WKO, et al. Team‐based approach reduces learning curve in robot‐assisted laparoscopic radical prostatectomy. Int J Urol 2006;13:560-4. [Crossref] [PubMed]
- Thinggaard E, Konge L, Bjerrum F, et al. Take-home training in a simulation-based laparoscopy course. Surg Endosc 2017;31:1738-45. [Crossref] [PubMed]
- Bric JD, Lumbard DC, Frelich MJ, et al. Current state of virtual reality simulation in robotic surgery training: a review. Surg Endosc 2016;30:2169-78. [Crossref] [PubMed]
- Kempenich JW, Willis RE, Campi HD, et al. The Cost of Compliance: The Financial Burden of Fulfilling Accreditation Council for Graduate Medical Education and American Board of Surgery Requirements. J Surg Educ 2018;75:e47-53. [Crossref] [PubMed]
- Lallas CD, Davis JWMembers Of The Society Of Urologic Robotic Surgeons. Robotic surgery training with commercially available simulation systems in 2011: a current review and practice pattern survey from the society of urologic robotic surgeons. J Endourol 2012;26:283-93. [Crossref] [PubMed]
- Chen R, Armijo PR, Krause C, et al. A comprehensive review of robotic surgery curriculum and training for residents, fellows, and postgraduate surgical education. Surg Endosc 2019; [Epub ahead of print]. [PubMed]
- Patel SV, Yu D, Elsolh B, et al. Assessment of conflicts of interest in robotic surgical studies: validating author's declarations with the open payments database. Ann Surg 2018;268:86-92. [Crossref] [PubMed]
- Perez RE, Schwaitzberg SD. Robotic surgery: finding value in 2019 and beyond. Ann Laparosc Endosc Surg 2019;4:51. [Crossref]
- deBeche-Adams T, Eubanks WS, Sebastian G. Early experience with the Senhance®-laparoscopic/robotic platform in the US. J Robot Surg 2019;13:357-9. [Crossref] [PubMed]
- Solinas M, Novellis P, Alloisio M, et al. Robotic innovations (instruments and new robots). Shanghai Chest 2018;2:68. [Crossref]
Cite this article as: Guenthart BA, Lui NS. Resident education in robotic thoracic surgery. Video-assist Thorac Surg 2020;5:18.