Anesthesia considerations for robotic thoracic surgery
Introduction
Over the past two decades, the popularity of robotic-assisted thoracic surgery has been on the rise. Common thoracic operations performed with robotic assistance include lung resection, diaphragmatic repair, esophagectomy, and resection of mediastinal tumors (1). Like other minimally invasive procedures, robotic-assisted surgery has the potential to decrease pain, blood loss and scarring. It may also reduce hospital length of stay in comparison with conventional open surgical procedures. A robotic system is superior to traditional video-assisted thoracoscopic surgery (VATS) because (I) it provides a three-dimensional view of the surgical field with magnification and (II) the articulating limbs of the robot allow micromanipulation and facilitate navigation in difficult to access spaces.
In particular for small lesions, mediastinal tumor resection using robotic-assisted VATS has been shown to be safe, technically easier to perform, and avoids a median sternotomy (2). Several recent case reports describe the use of robotic technology for the minimally-invasive resection of mediastinal masses which would not have been possible with traditional rigid-shaft VATS instruments (3-5). Although the robotic approach can increase surgical time, single center case reports indicate that potential benefits over VATS include decreased bleeding and increased yield in lymph node dissection (6).
The Da Vinci Robotic Surgical System (Intuitive Surgical Inc. Sunnyvale, CA, USA) is the most widely used robotic platform currently in use (Figure 1). Medtronic has recently revealed a new robot-assisted surgery platform anticipated to be available in the fall of 2019 (7). For the anesthesiologist, the management of a patient undergoing robotic-assisted thoracic surgery are similar to that of VATS, with some differences. One big difference arises in the bulkiness of the robot technology which can make anesthetic management complex. Here, we will review the anesthetic implications of robotic-assisted thoracoscopic surgery.
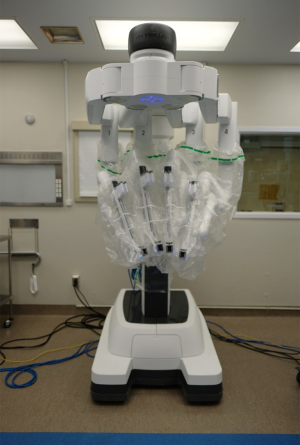
Pre-anesthesia evaluation
The basic pre-anesthesia evaluation for patients undergoing robotic thoracic surgery is similar to patients undergoing open or VATS procedures. A patient who is high risk for open thoracic surgery is also high risk for robotic surgery. In addition to the standard pre-anesthesia assessment, particular attention should be paid to the pulmonary, cardiac, and airway exam. In patients with lung cancer, another consideration is for side effects from chemotherapy or radiation. Basic metabolic panel (BMP), complete blood count (CBC), and blood type and screen are also obtained on all patients.
Patients being considered for thoracic surgery should undergo pulmonary function test (PFT) and preoperative cardiac evaluation (8). The American College of Chest Physicians (ACCP) recommends that initial testing should include spirometry with forced expiratory volume in 1 second (FEV1) and diffusing capacity of the lung for carbon monoxide (DLCO) (9). Patients with FEV1 >80%, no dyspnea on exertion, or DLCO >80% can proceed to surgery without further testing, otherwise the postoperative pulmonary reserve should be calculated. If the predicted postoperative pulmonary reserve of FEV1 or DLCO are found to be <40%, additional functional testing is recommended. These tests can include 10 minutes walk test, peak oxygen consumption (VO2), or ventilation-perfusion scintigraphy for large resections and pneumonectomy. The presence of baseline hypercapnia (PaCO2 >50 mmHg), hypoxemia (PaO2 <65 mmHg), abnormal PFTs, or pre-existing pulmonary disease such as moderate to severe chronic obstructive pulmonary disease (COPD) or emphysema (with FEV1 <50%) are all predictors to suggest that the patient will not be able to tolerate prolonged one-lung ventilation (OLV) or chest cavity CO2 insufflation. Patients with pulmonary hypertension or congestive heart failure may also have difficulty tolerating changes in venous return with insufflation (8). All patients should be counseled regarding smoking cessation.
Preoperative cardiac evaluation should include at least an electrocardiogram (ECG). A transthoracic echocardiogram can be considered if the patient has any risk factors such as a murmur, angina, or decreased exercise tolerance. Right ventricular (RV) dysfunction is common in COPD patients, and they may be poorly tolerant of sudden increases in afterload. Prior cardiac surgery or stents are not strict contraindications, but any patient with recent myocardial infarction should be seen and optimized by a cardiologist (10).
Appropriate patient selection is essential for success of robotic surgery. Severe cardiac and pulmonary disease should be considered contraindications to robotic surgery as these patients will not tolerate OLV or changes in venous return. Patients with a small thoracic cavity (height <130 cm) or body mass index greater than 35 kg/m2 can make endoscopic surgery visualization difficult. Additionally, prior thoracic surgery, history of thoracic trauma, or chest radiation may result in adhesions making the procedure technically very difficult (11).
Room layout
The operating surgeon is seated at a console several feet away from the patient. The surgical assistant stands to one side of the patient to assist with placement of trocars, changing the robotic instruments, and manipulating additional endoscopic instruments as needed. The scrub technician and instrument trays are located on the opposite side of the assistant surgeon. The anesthesiologist and anesthesia machine remain at the head of the patient (Figures 2,3).
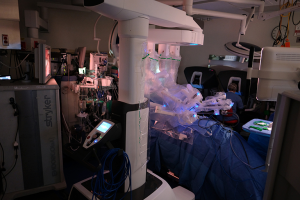
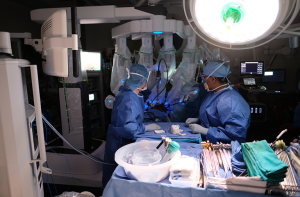
Monitoring and lines
In addition to routine monitoring recommended by the American Society of Anesthesiologists (ASA)-5-lead ECG, pulse oximetry, end-tidal CO2, temperature and blood pressure, we recommend placement of an arterial line for beat-to-beat blood pressure monitoring and regular arterial blood gas measurements. While there is generally minimal blood loss, at least two large-bore intravenous (IV) access lines are maintained in the event of needing fluid or blood transfusion, as positioning during these cases can render obtaining intra-operative IV access challenging. Urinary catheter insertion should also be considered for procedures of longer duration (>2 hours). Train-of-four monitoring is recommended to monitor adequate paralysis throughout the surgery. The authors of this paper do not routinely use central venous access unless there is significant cardiac comorbidity or difficulty with peripheral IV access.
Positioning and access
Careful attention must be paid to patient positioning in order to enable optimal surgical access and also prevent patient injury. Some procedures, such as esophagectomy and bilateral approach to the mediastinal lesions, may require multiple position changes, increasing the risk of patient injury.
Lateral decubitus
This is the most common position for robotic lung resection, diaphragmatic repair and the thoracic portion of esophagectomy.
Anesthesia is induced in the supine position. Once the airway is secured, the patient is transferred to the lateral position. Adequate operating room staff should be available to assist with positioning to ensure patient as well as personnel safety, especially in patients with morbid obesity or an unstable spine. The patient is positioned in a maximally flexed lateral decubitus position with the table break at the mid-thorax to maximize rib spaces (Figures 4,5). A gel roll anterior and posterior to the patient can be used to stabilize the patient in the lateral position. Alternatively, a beanbag is inflated to support the patient both anteriorly and posteriorly, and a hip strap applied to secure the patient. An axillary roll can be placed under the axilla to elevate the axillary space and prevent compression of the underlying neurovascular bundle (12).
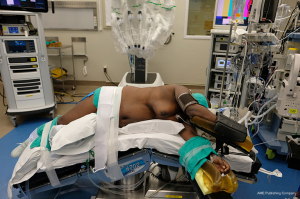
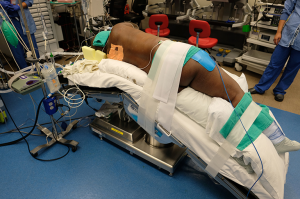
All pressure points such as elbows, knees and hips are padded with gel and foam pads. The arms are extended outwards parallel to each other. The lower arm is extended outward with the elbow slightly flexed. The upper arm is placed in a gel cradle on an airplane fixture, with the shoulder and elbow slightly flexed. The patient’s bottom leg is flexed, and the top leg kept straight with a pillow between the legs to prevent injury particularly to the common peroneal and saphenous nerve. Patient’s head should be in a neutral position with normal anatomical alignment with the rest of the body to avoid brachial plexus injury (12,13).
The lateral position is associated with the highest number of ocular complications, most frequently corneal abrasions (12,14). Eyes should be protected prior to positioning to avoid injury. Providers should take care to avoid external pressure on the dependent eye, and make sure the dependent ear is not bent, which can lead to ischemia (15).
Supine with tilt
This position is commonly used for approach to mediastinal structures. After securing the airway following induction of anesthesia, the operative side of the patient is tilted up about 30 degrees with a gel roll placed under the chest and shoulder. The lower arm may be tucked next to the body or extended on an arm board in a gel cradle with the elbow slightly flexed. The upper arm is allowed to drop below the body level on to another padded arm board next to the body with the shoulder partly extended to allow access to the chest. Here it is important to prevent brachial plexus injury from over-extension at the shoulder and to ascertain that the arm is supported and not hanging free.
Access to the patient
Once the robot is docked access to the patient can be limited. This means all vascular lines and airway tubes need to have adequate length and have proximal access points before the docking. The lung isolation technique should be reliable and positioning of the double-lumen tube (DLT) confirmed with a fiberoptic bronchoscope before docking the robot. The operating table cannot be moved without undocking the robot.
Once the patient is satisfactorily positioned, the anesthesia team and nursing staff should continue to check and re-evaluate patient positioning throughout the procedure. With the bulkiness of the robot, care must be taken to guard the patient against inadvertent contact of the robotic arms with the patient’s face or extremities (16). Any portion of the patient body that may come in contact with robotic arms needs to be protected with gel pads or sponge pads and constantly monitored to prevent injury from the large forces used to move the robot arms.
Intra-operative management
General anesthesia is induced and maintained by institutional and provider preference. Both total intravenous anesthesia (TIVA) and inhaled anesthetics are acceptable strategies for the maintenance of anesthesia. Nitrous oxide (N2O) is generally avoided. Complete muscle relaxation is recommended, as patient coughing and movement can have serious consequences when the robot is docked. The robot does not adjust to patient movement, and thus sudden patient movement can result in tissue injury and significant bleeding.
Lung isolation
Lung isolation is needed in order to facilitate visualization in the thoracic cavity. The choice of lung isolation technique should be according to the location of surgery and expertise of provider. Most commonly, a left-sided DLT is used for robotic cases because the left main bronchus is longer (average 4–5 cm) providing a greater margin of safety and more reliable lung collapse (17). Bronchoscopic confirmation of device placement with a fiberoptic scope is highly recommended both before and after positioning as lung isolation is critical to the facilitation of robotic procedures. Another option for lung isolation is the bronchial blocker. The most common bronchial blockers currently available include the Arndt, Cohen, Fuji and EZ Blocker. Each comes with its own benefits and drawbacks. In general, for robotic procedures, bronchial blockers are less commonly used as they can be more susceptible to migration and herniation into the trachea with position changes.
CO2 insufflation
CO2 insufflation is often used in minimally invasive procedures like laparoscopy and VATS. It acts as a tissue retractor, expanding the surgical field to allow better visualization of operative structures. Most centers commonly use low flows of about 5–10 L/min to insufflation pressures between 5 and 10 mmHg (11). However, this can have several deleterious effects on the patient that the anesthesiologist must be aware of.
Venous gas embolism
This has been described in both laparoscopic and robotic literature (18,19). It has not yet been reported in VATS or robotic-assisted VATS literature probably because the CO2 insufflation is often initiated through a port that is already visually established in the pleural cavity.
Tension capnothorax
A rare but potentially fatal complication of sudden CO2 insufflation in the chest cavity is tension capnothorax. Compression of mediastinal vessels can lead to hypotension which may need to be treated with IV fluids and vasoconstrictors. Stretching of the pleura can increase vagal tone causing severe bradycardia needing anticholinergic drugs. The compression of vascular structures causing hypotension and decreased cardiac output has been shown to be more prominent when the right chest is insufflated. In either event, decompression of the pleural cavity should be the important first step until the situation is under control (20). It can be prevented by making the insufflation more gradual-starting the CO2 flow at 5 L/min and a pressure of 5 mmHg and gradually building up to 10 mmHg.
Cardiovascular effects
Compression of the mediastinal structures like the atrium and vena cava can cause decreased venous return and decreased cardiac output (21). This has also been shown to be more pronounced with insufflation of the right chest. There can also be a transient increase in central venous pressure, pulmonary artery pressure and pulmonary artery wedge pressures.
Pulmonary effects
Insufflation of CO2 leading to a rise in PaCO2, has been shown to decrease pulmonary compliance (22). It can also increase airway pressures and decrease tidal volumes resulting in hypercarbia (16,23). As long as the patient does not have severe comorbidities, permissive hypercapnia is tolerated in order to maintain a protective lung ventilation strategy during OLV. Due to absorption of CO2 over time minute ventilation may need to be increased to eliminate this CO2 even after allowing for permissive hypercapnia. When the respiratory acidosis leads to a pH below approximately 7.25 the insufflation may need to be paused because this can affect, among other things, effective action of drugs such as inotropes.
Close communication between the surgical and anesthesia teams is required. Insufflation may need to be suddenly decreased or stopped if there are significant hemodynamic changes. An option is to continue using the robot without insufflation if it is poorly tolerated, but this limits visibility in the field, and both teams should be prepared to convert to an open procedure.
Ventilation
The duration of OLV should be minimized as much as possible. A retrospective case series by Licker et al. suggests that OLV greater than 100 minutes is associated with an increased risk for postoperative lung injury (18). Two-lung ventilation in the lateral position leads to several physiological changes. The non-operative lung is under-ventilated and over-perfused, while the operative lung is over-ventilated and under-perfused, resulting in V/Q mismatch. Additionally, the pressure from the mediastinum and the abdominal organs cause a decrease in functional residual capacity (FRC) and decreased compliance in the non-operative lung, and this is further exacerbated by insufflation of the operative side (12,15).
Once the operative side lung is deflated, hypoxic pulmonary vasoconstriction (HPV) plays an important role in preventing hypoxia by reducing perfusion to poorly oxygenated tissue (23). Vasoconstriction occurs in seconds and reaches an initial plateau; however, maximal response is only reached at 4 hours. HPV reduces shunt flow through the operative lung by up to 40%. Importantly, it can be inhibited by some inhalational agents, particularly N2O and the older inhalational agents ether and halothane. Clinically relevant concentrations of the newer inhaled anesthetics-desflurane, isoflurane and sevoflurane, as well as propofol appear to be neutral towards HPV (15).
Current evidence suggests that several strategies should be employed to protect against acute lung injury after lung resection and OLV. This includes low tidal volume ventilation (2–4 mL/kg), routine use of positive end-expiratory pressure (PEEP) to the non-operative lung, low ventilatory pressures, and permissive hypercapnia (19). To maintain adequate oxygenation during OLV a FiO2 of 1.0 is used for at least half an hour before introducing small amounts of air to reduce the FiO2 and decrease oxygen-related lung injury. This is to accommodate for the biphasic nature of HPV.
In patients with pre-existing cardiac or respiratory comorbidities, inadequate ventilation can result in severe respiratory acidosis, worsening pulmonary hypertension and RV dysfunction. If this occurs, the patient may need to be switched to higher tidal volume-low ventilation. If this is still poorly tolerated, the surgeon and anesthesiologist should discuss converting to an open procedure which would facilitate intermittent two-lung ventilation. Whenever possible, efforts should be made to extubate the patient in the operating room at the end of the procedure.
Hypoxia during OLV
With improving patient selection, advanced airway management, and better understanding of anesthetic effects, the incidence of intraoperative hypoxemia during OLV is declining, with some centers reporting the incidence of intraoperative hypoxia as low as 1% (24). However, with hypoventilation secondary to lung-protective ventilation strategies as well as decreased FiO2 administration to reduce incidence of acute lung injury, hypoxia can still occur. Predictors of decreased intraoperative PaO2 include: supine position, right-sided surgery, low PaO2 during two-lung ventilation in the lateral position, increased perfusion to the operative lung on pre-operative lung perfusion studies, body mass index (BMI) >30 kg/m2, and prior lobectomy (25). Strategies to address mild hypoxemia include: Confirm FiO2 is 1.0, confirm position of lung isolation device with the fiberoptic scope, and recruit and optimize PEEP in the non-operative lung. Other options such as continuous positive airway pressure (CPAP) to the operative lung after recruitment or intermittent two lung ventilation cannot be utilized during robotic-assisted procedures. Application of a pulmonary artery clamp by the surgical team to further reduce blood flow to the non-operative lung is occasionally done in the setting of severe hypoxemia in open thoracotomy or VATS, but this is difficult to do with the robot. In severe, refractory hypoxemia, the robotic approach needs to be abandoned and two lung ventilation with 100% oxygen should be resumed.
Analgesia
The use of robotic techniques enables the procedure to be done through a few small incisions, eliminating the need for a thoracotomy or midline sternotomy. This significantly reduces the analgesic requirement compared with open procedures, however, preliminary studies suggest mixed differences in pain scores between the robotic vs. non-robotic minimally invasive approach (26,27). Multimodal analgesia is utilized to reduce opioid requirements, encourage immediate extubation in the operating room, and avoid post-operative hypoventilation. While thoracic epidurals are done at some centers for minimally-invasive cases, intercostal nerve blocks are a simple procedure done under direct visualization by the surgical team at the end of the case that are also effective. Other regional techniques growing in popularity with the option for unilateral pain control also include paravertebral blocks and erector spinae plane blocks. For patients on anticoagulation, the guidelines set forth by the American Society of Regional Anesthesia are followed for neuraxial procedures (28).
Complications
Issues can arise that are the same as all OLV procedures, but some issues unique to robotic procedures such as tension capnothorax are described above. The anesthesiologist must always be prepared for the possibility of converting to an open procedure, and there should be a well-defined protocol in place for rapid undocking of the robot in the event of emergency. Thoracic procedures also have a higher incidence of arrhythmia, as well as hemodynamic instability on insufflation.
Conclusions
The popularity of robotic-assisted thoracic surgery is on the rise. Ongoing advances in robotic technology continue to rapidly expand the scope of procedures that can be done successfully. A steep learning curve exists for proficiency in robotic surgery, which can affect operating times and complication rates. Additionally, robotic thoracic surgery is currently more expensive than other options, but this is expected to be compensated for by decreasing length of hospital stay. In experienced hands, there are many potential advantages to robotic surgery, which hopefully include better patient outcomes as well as patient satisfaction. It is important for anesthesiologists to be familiar with the robotic system, understand aspects of perioperative management unique to these patients, and be aware of complications that can occur in order to ensure safe patient care.
Acknowledgments
We are grateful to Dr. P. Wang MD, for the photographs and to Dr. J. Brodsky MD for assistance with editing this manuscript.
Funding: None.
Footnote
Provenance and Peer Review: This article was commissioned by the Guest Editors (Natalie S. Lui and Sean C. Wightman) for the series “Robotic Surgery for Lung Cancer” published in Video-Assisted Thoracic Surgery. The article has undergone external peer review.
Conflicts of Interest: Both authors have completed the ICMJE uniform disclosure form (available at http://dx.doi.org/10.21037/vats.2019.12.08). The series “Robotic Surgery for Lung Cancer” was commissioned by the editorial office without any funding or sponsorship. The authors have no other conflicts of interest to declare.
Ethical Statement: The authors are accountable for all aspects of the work in ensuring that questions related to the accuracy or integrity of any part of the work are appropriately investigated and resolved.
Open Access Statement: This is an Open Access article distributed in accordance with the Creative Commons Attribution-NonCommercial-NoDerivs 4.0 International License (CC BY-NC-ND 4.0), which permits the non-commercial replication and distribution of the article with the strict proviso that no changes or edits are made and the original work is properly cited (including links to both the formal publication through the relevant DOI and the license). See: https://creativecommons.org/licenses/by-nc-nd/4.0/.
References
- Campos JH. An update on robotic thoracic surgery and anesthesia. Curr Opin Anaesthesiol 2010;23:1-6. [Crossref] [PubMed]
- Straughan DM, Fontaine JP, Toloza EM. Robotic-assisted videothoracoscopic mediastinal surgery. Cancer Control 2015;22:326-30. [Crossref] [PubMed]
- Nardini M, Dunning J, Migliore M, et al. Robotic resection of a middle mediastinal mass. J Vis Surg 2018;4:113. [Crossref] [PubMed]
- Park SY, Kim HK, Jang DS, et al. Initial experiences with robotic single-site thoracic surgery for mediastinal masses. Ann Thorac Surg 2019;107:242-7. [Crossref] [PubMed]
- Yang HC, Coyan G, Vercauteren M, et al. Robot-assisted en bloc anterior mediastinal mass excision with pericardium and adjacent lung for locally advanced thymic carcinoma. J Vis Surg 2018;4:115. [Crossref] [PubMed]
- Deng HY, Huang WX, Li G, et al. Comparison of short-term outcomes between robot-assisted minimally invasive esophagectomy and video-assisted minimally invasive esophagectomy in treating middle thoracic esophageal cancer. Dis Esophagus 2018; [Crossref] [PubMed]
- Kelly S. Medtronic expects revenue from surgical robot in fiscal 2019. Technology News 2016. Available online: https://www.reuters.com/article/us-medtronic-robot/medtronic-expects-revenue-from-surgical-robot-in-fiscal-2019-idUSKCN0YS2C3
- Slinger P, Campos J. Anesthesia for thoracic surgery. In: Miller R. editor. Miller’s anesthesia. 8th ed. Philadelphia: Saunders, 2014:1942-2107.
- Colice GL, Shafazand S, Griffin JP, et al. Physiologic evaluation of the patient with lung cancer being considered for resectional surgery: ACCP evidenced-based clinical practice guidelines (2nd edition). Chest 2007;132:161S-77S.
- British Thoracic Society, Society of Cardiothoracic Surgeons of Great Britain and Ireland Working Party. BTS guidelines: guidelines on the selection of patients with lung cancer for surgery. Thorax 2001;56:89-108. [Crossref] [PubMed]
- Bernstein WK, Walker A. Anesthetic issues for robotic cardiac surgery. Ann Card Anaesth 2015;18:58-68. [Crossref] [PubMed]
- Knight DJW, Mahajan RP. Patient positioning in anaesthesia. Continuing Education in Anaesthesia, Critical Care & Pain 2004;4:160-3. [Crossref]
- Cassorla L, Lee J. Patient positioning and associated risks. In: Miller R. editor. Miller’s anesthesia. 8th ed. Philadelphia: Saunders, 2014:1445-71.
- Roth S, Thisted RA, Erickson JP, et al. Eye injuries after nonocular surgery. A study of 60,965 anesthetics from 1988 to 1992. Anesthesiology 1996;85:1020-7. [Crossref] [PubMed]
- Chaverneff F. Patient positioning during anesthesia: lateral decubitus. Clin Pain Adv 2019.
- Lee JR. Anesthetic considerations for robotic surgery. Korean J Anesthesiol 2014;66:3-11. [Crossref] [PubMed]
- Campos J, Ueda K. Update on anesthetic complications of robotic thoracic surgery. Minerva Anestesiol 2014;80:83-8. [PubMed]
- Licker M, de Perrot M, Spiliopoulos A, et al. Risk factors for acute lung injury after thoracic surgery for lung cancer. Anesth Analg 2003;97:1558-65. [Crossref] [PubMed]
- Lohser J. Evidence-based management of one-lung ventilation. Anesthesiol Clin 2008;26:241-72. [Crossref] [PubMed]
- Pauli H, Eladawy M, Park J. Anesthesia for robotic thoracic surgery. Ann Cardiothorac Surg 2019;8:263-8. [Crossref] [PubMed]
- Ohtsuka T, Nakajima J, Kotsuka Y, et al. Hemodynamic responses to intrapleural insufflation with hemipulmonary collapse. Surg Endosc 2001;15:1327-30. [Crossref] [PubMed]
- El-Dawlatly AA, Al-Dohayan A, Abdel-Meguid ME, et al. Variations in dynamic lung compliance during endoscopic thoracic sympathectomy with CO2 insufflation. Clin Auton Res 2003;13:I94-7. [Crossref] [PubMed]
- El-Dawlatly AA, Al-Dohayan A, Samarkandi A, et al. Right vs left side thoracoscopic sympathectomy: effects of CO2 insufflation on haemodynamics. Ann Chir Gynaecol 2001;90:206-8. [PubMed]
- Brodsky JB, Lemmens HJ. Left double-lumen tubes: clinical experience with 1,170 patients. J Cardiothorac Vasc Anesth 2003;17:289-98. [Crossref] [PubMed]
- Campos JH, Feider A. Hypoxia during one-lung ventilation-a review and update. J Cardiothorac Vasc Anesth 2018;32:2330-8. [Crossref] [PubMed]
- Molinaro F, Krasniqi P, Scolletta S, et al. Considerations regarding pain management and anesthesiological aspects in pediatric patients undergoing minimally invasive surgery: robotic vs laparoscopic-thoracoscopic approach. J Robot Surg 2019; [Epub ahead of print]. [Crossref] [PubMed]
- van der Ploeg APT, Ayez N, Akkersdijk GP, et al. Postoperative pain after lobectomy: robot-assisted, video-assisted and open thoracic surgery. J Robot Surg 2019; [Epub ahead of print]. [PubMed]
- Horlocker TT, Vandermeuelen E, Kopp SL, et al. Regional anesthesia in the patient receiving antithrombotic or thrombolytic therapy: american society of regional anesthesia and pain medicine evidence-based guidelines (fourth edition). Reg Anesth Pain Med 2018;43:263-309.
Cite this article as: Ruan A, Kulkarni V. Anesthesia considerations for robotic thoracic surgery. Video-assist Thorac Surg 2020;5:6.