Regional lung chemotherapy techniques to enable or enhance minimally invasive lung surgery
Introduction
Because of the related technologies that thoracic surgeons use to care for their patients during and after surgery, it is not surprising that there is a long history of attempting regional lung chemotherapy for pulmonary malignancy. For instance, the pulmonary artery catheter made it possible to select one lung or another for study and Karakousis et al. used this in 1981 to target chemotherapy for sarcoma (1). The heart-lung machine provided the ability to recirculate and oxygenate the systemic circulation. Creech applied this to the lung similarly in 1959 (2). About 25 years later, investigators employed this methodology to perfuse human lung with chemotherapy clinically (3,4). Enthusiasm for such targeted methods described in this review was tempered by inconsistent or inadequate results and relatively high technique-associated morbidities. These results have also been diminished by our relatively poor understanding of patient-specific tumor biology and host immune dynamics and, in some ways, mirrored some of the failures of promising systemic chemotherapy. Unlike systemic chemotherapy, clinical research for surgical targeted chemo was slowed by the relative expense of the operating room and the delivery technologies involved.
Over this same time that regional lung chemotherapy has struggled to make its mark, there has been steady improvement in the ability of surgeons to confront even advanced malignancy by minimally invasive techniques. Thus, overall video-assisted thoracoscopic surgery (VATS) lobectomy reliability rates have exceeded 90% signaling inclusion of advanced cancer patients who realize many of the benefits seen for earlier stage disease (5-7). There may be additional benefits in that the better preservations of host performance and reductions in blood loss and opioid requirements enabled by less invasive surgery allow for fewer disruptions of essential systemic therapies and innate immune responses. Furthermore, local therapies like surgery or radiation should probably strive for even less toxicity as their relative anticipated benefits diminish inversely with cancer stage.
Some stage III tumors demonstrate promising partial responses with induction therapies to clinical situations that have enabled minimally invasive, rather than open, approaches. However, disease stability or progression that requires conversion or planned open thoracotomy keep VATS reliability short of 100%. The therapies described in this article could be of interest to surgeons striving to reach an even higher goal for their lung resection program by enabling even better primary tumor responses. Even more excitingly, the expansion of imaging, pharmacologic and perfusion technologies used by our colleagues in medicine, diagnostic and therapeutic radiology, cardiac surgery, lung transplantation, and others have created exciting opportunities for thoracic surgeons to help enhance current operations and even extend them to groups we have not been able to help, like stage IV patients. Newer immune-based therapies are aided by interventions directed selectively to the bulk of disease to generate an “abscopal” effect toward the rest of systemic disease (8).
This article will review contemporary methods (largely done as part of clinical trials) to achieve regional lung therapy and will briefly describe the aspects of the lung anatomy and physiology that make this a promising organ on which to expand such efforts. Broader and historical reviews on this topic by Mallick and others are available to the reader (9,10).
Pulmonary circulation
The minute cardiac output approximates the blood volume that is about 5 liters. The pulmonary component is about 10% of the blood volume yielding about 250 mL per lung that is targeted. The lung vasculature is a low pressure and high flow system with a rich, high-compliance network (30 mL/mmHg) that holds about a third of the blood volume and has the ability to easily distend and recruit unused capillary beds (11). Thus, the pulmonary resistance actually falls with increasing intravascular pressure.
The lung circulation (arteries and veins) and microcirculation (<50 µM) create a complex sinusoidal anatomy, the physiology of which has been difficult to study in a normal subject much less in the perturbed state of malignancy. Many factors can affect flow and perfusion such as gravity (e.g., West zones), airway closure or tethering open of small vessels, and cytokine-induced venous constriction (9).
Often ignored, because it contributes only about 1% of the pulmonary blood flow in non-pathologic states, is the bronchial artery component. This becomes important in pathologic states such as central lung tumors or bronchiectasis (up to 30% of cardiac output) or in situations where small contributions over time become problematic such as attempts to isolate the lung for regional lung chemotherapy (12). Unfortunately, the anatomy of the bronchial arteries is quite variable with half of patients having a single vessel on one side and two on the other (13). Typically, these 1.5–2 mm vessels arise from the anterior descending aorta at T5–6 level and terminate at the capillary level within the pulmonary circulation. Manipulating or perfusing them can be hazardous because of collateral vessels that feed unforgiving organs such as the spinal cord or esophagus. Other regional arteries spanning from the chest (e.g., internal thoracic) to the abdomen (e.g., left gastric) can supply the bronchial circulation as well.
With respect to injury, the lung is a delicate organ both anatomically and physiologically and this is the primary concern with interventions that target it. That stated, it has certain characteristics and tolerances that make it ideal for some of the methods described in this article. First, the high degree of vascular interconnectivity described above is useful for delivery of agents instilled into any of the vessel lumens. Next, the lung tolerates deflation for extended times without injury (14). Finally, the alveolar epithelium tolerates hypoxia quite well and lung can maintain its function reasonably well despite upregulation of pro-inflammatory cytokines (15,16).
Intravascular and airway techniques for localized delivery of chemotherapeutic agents
The methods used for lung regional therapy are classified broadly into single injection or single pass techniques and those that require an extracorporeal system to perfuse the organ from a reservoir containing the desired drug. Results from such clinical studies are difficult to interpret because patients go on to receive systemic or other local therapies such as surgery and radiation. In general, indications for localized therapy are broad and generally require all or the majority of the measurable disease to exist in the targeted lung. Pregnancy, breast-feeding, allergy to the contrast media or desired agent are relative contraindications. Side effects or complications common to all the treatments are fevers, chest pains, cough, hemoptysis, vomiting, transient hypotension or other hemodynamic changes, and hematoma at the site of percutaneous puncture.
Single pass based methods
Inhaled and direct tumor injection
With up to 100 m2 of epithelial surface area for absorption, it is understandable why the airways and alveoli would be promising areas for drug delivery. In addition, adding carbon dioxide to the aerosol increases deposition. The difficulty comes with preparing particles to be within a precise range of 1–3 µm to be able to reach the alveoli for deposition. This generally requires use of dry powder inhalers and nebulizers that generally deliver only about one-fourth of the agents to the lung and retain a similar amount within the devices (12). There is also the need for protective strategies for health-care personnel who might inhale these agents inadvertently.
There are differences in delivery based on whether the drugs are hydrophobic, hydrophilic, or engineered to have enhanced efficiency by nanotechnology. Some of these nanotechnology methods package drug by including linkage or incorporation into dozens of technologies that involve liposomes, microparticles, carbohydrates, polymers, bioadhesives, and cell-type targeting (12). In addition, the rate by which these strategies work depends on the location within the airway (Figure 1).
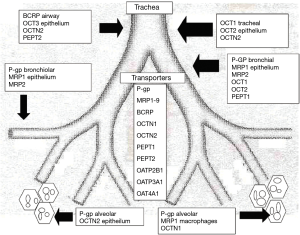
Human trials are limited but attempts were made with drugs like 5-FU, 9-nitro-camptothecin, carboplatin, cisplatin, doxorubicin, and gemcitabine (12). Respiratory inflammatory complications with reductions of PFTs >20% are the biggest problem with this strategy. In addition, one of the main barriers to its use for advanced tumors is the size limitation of the primary to be less than 5 cm. Therefore, while it is unlikely that this technology can shrink a large tumor to allow VATS, it was included in this article because of its potential to treat widespread smaller tumors or minimal residual disease. As the vascular methods described later, concomitant lung surgery also offers unique opportunities for selective and controlled lung ventilation to harness these technologies in ways not possible easily outside of the operating room.
Direct tumor injection using a transbronchial aspiration needle is another airway-based method to augment the local effects of systemic chemotherapy. The desired drug is delivered on an ml-to-ml basis to tumor volume using the formula volume =0.5 × height × width × depth using the maximal length in each dimension (17). Downstream injection leakage is monitored by mixing indigo carmine with the agent. Enhancing its practice has been the emergence of technologies such as endobronchial ultrasound to target involved lymph nodes and tumor and navigational bronchoscopy for more peripheral lesions. Bolus injection dispersion might be erratic based on the variations of intratumoral interstitial fluid pressure, hypoxia, vascular architecture, extracellular matrix, and gross tumor structure (17). Some of the same methodologies to concentrate and enhance local delivery for inhaled drugs are adaptable to injected agents. Notably, downstream leaked drug that fails direct absorption may still be useful if taken up by surrounding alveoli and lymphatics (Figure 2). This technique may be less toxic than inhaled methods and has demonstrated a remarkable local airway regression that could simplify an open or VATS bronchoplastic procedure (Figure 3). However, because patients also received systemic therapy a precise attribution is not possible.
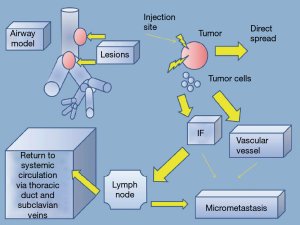
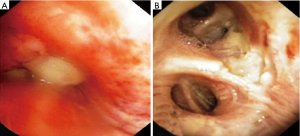
Arterial chemoembolization
Chemoembolization is a successful option for hepatic malignancies and is under study now for lung tumors. The technique begins by targeting the upstream segmental pulmonary artery branch similar to that used by Karakousis et al. who attempted to treat metastatic sarcoma without limiting antegrade flow (Figure 4). Once targeted, balloon occlusion interrupts inflow while chemotherapeutic agents like mitomycin C and flow arrestors like iodized oil and microspheres are delivered to the tumor (18). Before deflating the balloon, permanent artery occlusions using coils or other agents, as well as temporary degradable occlusion by starch or gelatin sponges prevent the drugs from washing away. About a third of patients experience tumor regression and there is interest in developing injectates that elute drug over a protracted time. Because there are potential distal bronchial artery collaterals from the pulmonary artery tree, paraplegia is a potential serious complication from this procedure.
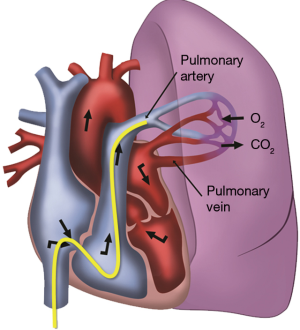
Bronchial artery infusion (BAI)
This method has developed especially well with the improved catheters available to interventional radiologists since its emergence over 50 years ago (19). Patients generally have multiple feeding bronchial arteries and about 25% have non-bronchial feeding vessels found by conventional angiographic techniques using superselective catheterization. Three-dimensional CT angiography was concordant with angiography in 70% of cases in 2013. Moreover, with better imaging technology, this rate will certainly improve (20). The best results occurred with multiple arterial infusions with combination agents (cisplatin, doxorubicin, and/or gemcitabine) where Nakanishi documented a 3% complete response and 50% partial response rate (21). In that work, cases that demonstrated high percentages (>75%) of total tumor blush achieved the best response rates (Figure 5). Patients received at least two treatments and they were staged 2–4 weeks apart with care taken to limit contrast medium to protect kidney function. The chemo was pushed slowly by hand with the catheter at the origin of the feeding artery so as not to occlude it. Some cases had dramatic responses and appear to sufficient to render a patient resectable by VATS, if appropriate (Figure 6) (22). The downside of this technique is that it is resource intensive, requires a lot of interventional radiologist expertise, and suffers from the unusual, but devastating potential complications of spinal cord injury or damage to other organs perfused by these vessels.
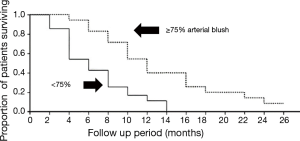
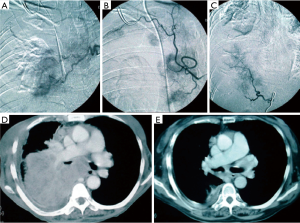
Lung suffusion
As the more complicated perfusion techniques described below, suffusion involves control of the pulmonary artery and veins; however, it does not employ their puncture and direct cannulation. Surgeons accomplished this by VATS control of the pulmonary veins using techniques learned from lobectomy. After their dissection and mobilization, silicone tapes double looped the veins to create a snare effect. Inflow control and distal drug delivery is by a transfemoral approach using a balloon catheter of sufficient girth to occlude the proximal right or left pulmonary artery. Given the wide range of minimally invasive approaches to the left atrium for the purpose of transcatheter valve or electrophysiological interventions, it is likely that the veins will be isolated percutaneously in the future.
Once vessels are controlled, the sequence in Table 1 is carried out to isolate the circulation and deliver chemotherapy to the lung. Figures 7-9 demonstrates the isolation strategy. This work emulates the cardiac vascular phenomenon by which an arterial dose of an organ preservation solution will fill the sinusoidal bed of the entire heart despite coronary occlusions if the venous drainage is impeded. Based on preclinical work, 75% of the drug remains locked in the desired lung for 30 minutes and slowly permeates (suffuses) the tissue uniformly (23). Also, preclinical work demonstrated an ablative effect on lymph nodes draining the target lung. The maximal tolerated dose in a canine model was 25% of a systemic dose (24).
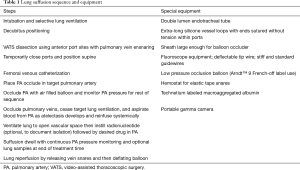
Full table
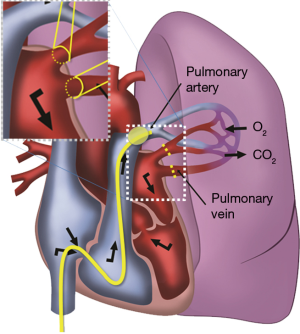
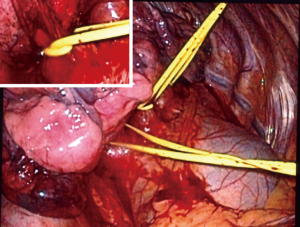
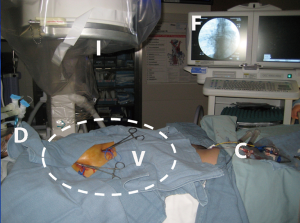
In clinical work, this technique was carried out successfully in 10 patients with a variety of pathologies including oligometastatic lung cancer and pulmonary metastases with an objective to determine the maximal tolerated dose in the normal tissue. One additional patient did not tolerate occlusion of the pulmonary vessels and did not receive the chemotherapy. So far, the patients (5% and 7.5% systemic) have experienced minimal low toxicity and have been discharged in 1–3 days to start additional therapies, if indicated, without delay (24). The dose level currently reached is 10% of a systemic dose. While not designed to determine outcomes, 7 patients had measurable disease after the suffusion. Of these, there was 1 complete response, 1 partial response, 1 stable disease (all difficult to distinguish from effect of sequential chemo) and, more importantly, 4 differential responses (suffused tissue stabilized or regressed while non-suffused metastases progressed).
The cost of the relatively expensive operating room environment needed to accomplish the suffusion if being done as a stand-alone procedure hampered its progress. It is relatively inexpensive if added onto an established operation like metastasectomy.
Recirculation based methods
Experimentally in small animal models, it is possible to emulate a recirculation-based system using a large quantity of perfusate and discarding it using a venotomy (25). In humans, this is not practical and generally, a recirculating perfusion system has been used. Unfortunately, some pulmonary toxicity has been attributed to these systems themselves in addition to the cytotoxic agents they contain. Recently, investigators harnessed gentler, more physiologic methods to perfuse donated lung organs ex vivo in order to resuscitate them (26). These same systems are being applied in promising preclinical work to eliminate the adverse effects of extracorporeal circulation on lung tissue.
Isolated lung perfusion (ILP)
Limb perfusion using a recirculating system is effective for tumors like melanoma and sarcoma (27). A typical system is demonstrated schematically in Figure 10. Other items that may be included in this circuit are a heat exchanger for warming the perfusate and a small reservoir to keep the prime volume low. An oxygenator is not needed because of the low metabolic needs of the lung and its ability to get oxygen by ventilation. Like BAI techniques, ILP was described over 50 years ago but was not attempted in humans until 1986 when it was associated with a high mortality (3). Since that time, six phase I trials averaging about 10 patients each have demonstrated much better safety but only modest evidence for tumor response (28-33). One of these trials had a long-term survival that was more promising which led to a multicenter Phase ILP trial of 50 patients with metastatic pulmonary sarcoma (12 with bilateral treatments) using 45 mg of melphalan in a 37 °C. perfusate for 30 minutes with a 5-minute washout (34,35). There was no operative mortality and a 57% overall 3-year survival. Like inhaled therapy, ILP resulted in a 20% reduction in pulmonary function. Although there was not a control group, investigators recommended further study based on favorable patient outcomes compared to historical results.
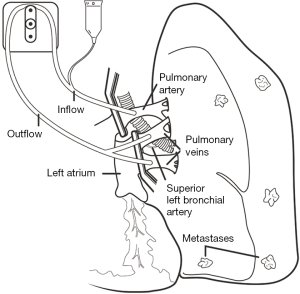
It is unclear whether an intended randomized Phase III trial for this technique of ILP will go forward. Because of the morbidity from the perfusion-driven cytokine and chemotherapeutic lung dysfunction and the thoracotomy required for cannulation in patients with advanced stages of cancer, a significant improvement in survival is required to increase its popularity. However, given improvements in catheter design, it is reasonable to believe that thoracoscopic ILP cannulation is possible and there has been preclinical work in this regard (36). Furthermore, many improvements in lung perfusion technology have occurred.
In vivo lung perfusion (IVLP)
Ex vivo perfusion, incorporating protective extracorporeal circulation features, actually improves the function of impaired donor lungs (26). This success logically leads investigators to bridge this same technology to avoid the previous problems with ILP lung injury caused more by the circuit biomaterials than the chemotherapy itself. Preclinical experiments (Figure 11) have been successful in prolonged lung perfusion with doxorubicin in vivo lasting 8 times longer (4 hours) than the typical 30-minute ILP used clinically (37). Previous experiments that showed that there was no decline in lung function from IVLP alone attributed this to the innovations listed in Table 2.
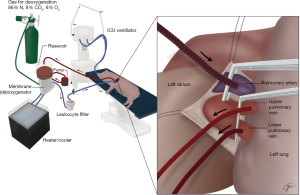
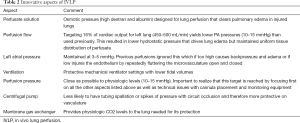
Full table
While no human trials for this strategy have been published yet, it is reasonable to expect that they are underway by the Toronto group that has made so many recent contributions to these and related technologies.
Technique selection and future trends
Changing normative practice in medicine can be a lengthy process even when there is evidence from well-designed randomized clinical trials. For patients with advanced malignancy where there are many competing technologies such as new targeted drugs directed to modulate patient-specific genomic finding that do not require invasive procedures, it is not surprising that many of the techniques described in this article have not had an increased popularity. However, technology to deliver targeted therapies has progressed nicely over the past decades and many patients will have an opportunity to benefit from them while simultaneously experiencing other diagnostic and staging procedures. This is provided their toxicity and invasiveness do not interfere with systemic therapies. Furthermore, it seems likely that they could be useful to prime local tumor responses as part of comprehensive immunotherapy treatment strategy.
The optimal technique for a patient will depend on the objective of local therapy (e.g., cytoreductive to reduce the toxicity of surgery or radiation, eradication of minimal residual disease, lymph node targeting, etc.) and the patient’s tumor anatomy and host physiologic reserve. It seems likely that these novel therapies will combine with traditional ones and be selected based on availability of technology and human resources. For instance, advanced interventional radiology experience might drive a BAI program, interventional pulmonary medicine directs tumor injection, and a lung transplant program in vivo perfusion. Table 3 lists the techniques described in this article and their relative strengths.
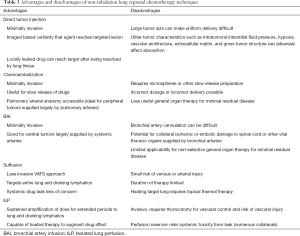
Full table
Future trends are likely to include less invasive ways of perfusing the desired portion of the lung using transseptal catheterization and incorporation of less injurious perfusion strategies as outlined in the in vivo perfusion section. Bronchial artery perfusion seems underutilized, especially for the 10% of patients whose lung tumors derive over 75% of their blood supply from systemic vessels. Better CT imaging advances will identify such patients more readily in the future. Enhanced targeting by navigational bronchoscopy or spin CT imaging in hybrid operating rooms will allow more directed tumor ablation after which anti-neoplastic therapy is administered to deal with minimal residual local disease beyond image resolution. Nanotechnology will continue to improve to allow better packaging of anti-tumor agents for increased delivery or long-term elution. It is also likely that successful aspects from other clinical regional programs such as heated intraperitoneal chemotherapy will be applied to lung tumors.
Conclusions
There has been a lot of interest in enhancing regional lung therapies to improve local control and possibly effect cure. Such treatments will continue to evolve and represent viable alternatives for patients with tumor biology too difficult to control or in whom frailty and comorbidities limit traditional options. Potential benefits of these therapies need to be weighed against the risks associated with their delivery. Inhaled agents are interesting but have respiratory toxicity and tumor size limitations. Direct injection of the tumor and involved lymph nodes with enhanced drugs will likely increase as enhanced and more accessible imaging enables more physician groups to participate. Vascular targeting of tumors is more complicated but avoids some toxicity of airway-based methods and has a better potential to treat large areas of the lung and draining lymphatics. Its future depends on simplifying and reducing the invasiveness the infusion or perfusion technologies associated with its use. Some of the treatments described might be useful for reducing the tumor size and invasion of structures difficult to resect surgically in order allow an R0 resection or enable a minimally invasive approach for patients too frail for thoracotomy.
Acknowledgments
Funding: None.
Footnote
Provenance and Peer Review: This article was commissioned by the editorial office, Video-Assisted Thoracic Surgery for the series “VATS for Locally Advanced Lung Cancer”. The article has undergone external peer review.
Conflicts of Interest: The author has completed the ICMJE uniform disclosure form (available at http://dx.doi.org/10.21037/vats.2018.04.07). The series “VATS for Locally Advanced Lung Cancer” was commissioned by the editorial office without any funding or sponsorship. TLD served as the unpaid Guest Editor of the series and serves as an unpaid editorial board member of Video-Assisted Thoracic Surgery from Apr 2017 to May 2019. The author has no other conflicts of interest to declare.
Ethical Statement: The author is accountable for all aspects of the work in ensuring that questions related to the accuracy or integrity of any part of the work are appropriately investigated and resolved.
Open Access Statement: This is an Open Access article distributed in accordance with the Creative Commons Attribution-NonCommercial-NoDerivs 4.0 International License (CC BY-NC-ND 4.0), which permits the non-commercial replication and distribution of the article with the strict proviso that no changes or edits are made and the original work is properly cited (including links to both the formal publication through the relevant DOI and the license). See: https://creativecommons.org/licenses/by-nc-nd/4.0/.
References
- Karakousis CP, Park HC, Sharma SD, et al. Regional chemotherapy via the pulmonary artery for pulmonary metastases. J Surg Oncol 1981;18:249-55. [Crossref] [PubMed]
- Creech O, Krementz ET, Ryan RF, et al. Experiences with isolation-perfusion technics in the treatment of cancer. Ann Surg 1959;149:627-40. [Crossref] [PubMed]
- Krementz ET. Lucy Wortham James lecture. Regional perfusion. Current sophistication, what next? Cancer 1986;57:416-32. [Crossref] [PubMed]
- Minchin RF, Johnston MR, Aiken MA, et al. Pharmacokinetics of doxorubicin in isolated lung of dogs and humans perfused in vivo. J Pharmacol Exp Ther 1984;229:193-8. [PubMed]
- Hennon M, Sahai RK, Yendamuri S, et al. Safety of thoracoscopic lobectomy in locally advanced lung cancer. Ann Surg Oncol 2011;18:3732-6. [Crossref] [PubMed]
- Pischik VG. Technical difficulties and extending the indications for VATS lobectomy. J Thorac Dis 2014;6:S623-30. [PubMed]
- Lee PC, Kamel M, Nasar A, et al. Lobectomy for non-small cell lung cancer by video-assisted thoracic surgery: Effects of cumulative institutional experience on adequacy of lymphadenectomy. Ann Thorac Surg 2016;101:1116-22. [Crossref] [PubMed]
- Lesueur P, Chevalier F, Stefan D, et al. Review of the mechanisms involved in the abscopal effect and future directions with a focus on thymic carcinoma. Tumori 2017;103:217-22. [Crossref] [PubMed]
- Mallick R, Demmy T. Regional lung chemotherapy techniques. Innovations (Phila) 2011;6:1-9. [Crossref] [PubMed]
- Ward A, Prokrym K, Pass H. Isolated lung perfusion for pulmonary metastases. Thorac Surg Clin 2016;26:55-67. [Crossref] [PubMed]
- Hughes JMB, Morrell NW. Pulmonary Circulation: From Basic Mechanisms to Clinical Practice. London: Imperial College Press, 2001.
- Zarogoulidis P, Chatzaki E, Porpodis K, et al. Inhaled chemotherapy in lung cancer: Future concept of nanomedicine. Int J Nanomedicine 2012;7:1551-72. [Crossref] [PubMed]
- Riquet M. Bronchial arteries and lymphatics of the lung. Thorac Surg Clin 2007;17:619-38. viii. [Crossref] [PubMed]
- Whittenberger JL, McGregor M, Berglund E, et al. Influence of state of inflation of the lung on pulmonary vascular resistance. J Appl Physiol 1960;15:878-82. [Crossref] [PubMed]
- Funakoshi T, Ishibe Y, Okazaki N, et al. Effect of re-expansion after short-period lung collapse on pulmonary capillary permeability and pro-inflammatory cytokine gene expression in isolated rabbit lungs. Br J Anaesth 2004;92:558-63. [Crossref] [PubMed]
- Jain M, Sznajder JI. Effects of hypoxia on the alveolar epithelium. Proc Am Thorac Soc 2005;2:202-5. [Crossref] [PubMed]
- Hohenforst-Schmidt W, Zarogoulidis P, Darwiche K, et al. Intratumoral chemotherapy for lung cancer: Re-challenge current targeted therapies. Drug Des Devel Ther 2013;7:571-83. [PubMed]
- Vogl TJ, Shafinaderi M, Zangos S, et al. Regional chemotherapy of the lung: Transpulmonary chemoembolization in malignant lung tumors. Semin Intervent Radiol 2013;30:176-84. [Crossref] [PubMed]
- Kahn PC, Paul RE, Rheinlander HF. Selective bronchial arteriography and intra-arterial chemotherapy in carcinoma of the lung. J Thorac Cardiovasc Surg 1965;50:640-5. [PubMed]
- Ye XD, Yuan Z, Ye JD, et al. Assessment of the feeding arteries by three-dimensional computed tomography angiography prior to multi-arterial infusion chemotherapy for lung cancer. Oncol Lett 2013;5:363-7. [Crossref] [PubMed]
- Nakanishi M, Demura Y, Umeda Y, et al. Multi-arterial infusion chemotherapy for non-small cell lung carcinoma--significance of detecting feeding arteries and tumor staining. Lung Cancer 2008;61:227-34. [Crossref] [PubMed]
- Nakanishi M, Umeda Y, Demura Y, et al. Effective use of multi-arterial infusion chemotherapy for advanced non-small cell lung cancer patients: Four clinical specified cases. Lung Cancer 2007;55:241-7. [Crossref] [PubMed]
- Demmy TL, Wagner-Mann C, Allen A. Isolated lung chemotherapeutic infusions for treatment of pulmonary metastases: A pilot study. J Biomed Sci 2002;9:334-8. [Crossref] [PubMed]
- Demmy TL. Thoracoscopic lung suffusion. Thorac Surg Clin 2016;26:109-21. [Crossref] [PubMed]
- Van Thielen J, Wittock A, Hendriks J, et al. Isolated lung perfusion with gemcitabine combined with radiotherapy: No additional lung toxicity in an experimental model. Eur J Cardiothorac Surg 2012;42:712-8. [Crossref] [PubMed]
- Van Raemdonck D, Neyrinck A, Cypel M, et al. Ex-vivo lung perfusion. Transpl Int 2015;28:643-56. [Crossref] [PubMed]
- Lienard D, Ewalenko P, Delmotte JJ, et al. High-dose recombinant tumor necrosis factor alpha in combination with interferon gamma and melphalan in isolation perfusion of the limbs for melanoma and sarcoma. J Clin Oncol 1992;10:52-60. [Crossref] [PubMed]
- Burt ME, Liu D, Abolhoda A, et al. Isolated lung perfusion for patients with unresectable metastases from sarcoma: A phase I trial. Ann Thorac Surg 2000;69:1542-9. [Crossref] [PubMed]
- Hendriks JM, Grootenboers MJ, Schramel FM, et al. Isolated lung perfusion with melphalan for resectable lung metastases: A phase I clinical trial. Ann Thorac Surg 2004;78:1919,26; discussion 1926-26; discussion 1926-7.
- Johnston MR, Minchen RF, Dawson CA. Lung perfusion with chemotherapy in patients with unresectable metastatic sarcoma to the lung or diffuse bronchioloalveolar carcinoma. J Thorac Cardiovasc Surg 1995;110:368-73. [Crossref] [PubMed]
- Pass HI, Mew DJ, Kranda KC, et al. Isolated lung perfusion with tumor necrosis factor for pulmonary metastases. Ann Thorac Surg 1996;61:1609-17. [Crossref] [PubMed]
- Ratto GB, Toma S, Civalleri D, et al. Isolated lung perfusion with platinum in the treatment of pulmonary metastases from soft tissue sarcomas. J Thorac Cardiovasc Surg 1996;112:614-22. [Crossref] [PubMed]
- Schröder C, Fisher S, Pieck AC, et al. Technique and results of hyperthermic (41 degrees C) isolated lung perfusion with high-doses of cisplatin for the treatment of surgically relapsing or unresectable lung sarcoma metastasis. Eur J Cardiothorac Surg 2002;22:41-6. [Crossref] [PubMed]
- Den Hengst WA, Van Putte BP, Hendriks JM, et al. Long-term survival of a phase I clinical trial of isolated lung perfusion with melphalan for resectable lung metastases. Eur J Cardiothorac Surg 2010;38:621-7. [Crossref] [PubMed]
- den Hengst WA, Hendriks JM, Balduyck B, et al. Phase II multicenter clinical trial of pulmonary metastasectomy and isolated lung perfusion with melphalan in patients with resectable lung metastases. J Thorac Oncol 2014;9:1547-53. [Crossref] [PubMed]
- Jinbo M, Ueda K, Kaneda Y, et al. Video-assisted transcatheter lung perfusion regional chemotherapy. Eur J Cardiothorac Surg 2005;27:1079-82. [Crossref] [PubMed]
- Reck Dos Santos P, Sakamoto J, Chen M, et al. Modified in vivo lung perfusion for local chemotherapy: A preclinical study with doxorubicin. Ann Thorac Surg 2016;101:2132-40. [Crossref] [PubMed]
Cite this article as: Demmy TL. Regional lung chemotherapy techniques to enable or enhance minimally invasive lung surgery. Video-assist Thorac Surg 2018;3:23.